Visomitin eye drops in the treatment of dystrophic changes in the anterior part of the eye. SKQ1 Mitochondria

A few years ago a small molecule SkQ1 was synthesized by the group of professor Vladimir P. Skulachev in the Moscow State University. One part of SkQ1 functions as a molecular “tow truck” carrying the other part of the molecule — an extremely active antioxidant plastoquinone — into mitochondria. Both theoretical calculations and experimental results showed that SkQ1 was delivered into the mitochondria in an extremely targeted and efficient manner. The physics of the mitochondrial membrane and the unique properties of SkQ1 direct it into the inner leaflet of the inner mitochondrial membrane with high precision.
The presence of SkQ1 in the mitochondrial membrane enables mitochondria to protect itself from reactive oxygen species (ROS) by breaking the chain reaction of lipid destruction. This ability of the lead molecule to protect cells against oxidative stress is the key to treating patients suffering from various age-related disorders such as cardiovascular diseases, neurodegenerative disorders, and ophthalmic conditions.
More than a dozen studies have been conducted and showed SkQ1 effectiveness in such critical therapeutic areas as the following:
- Age-related macular degeneration (AMD)
- Dry eye syndrome (DES)
- Non-infectious uveitis
- Cataract
- Glaucoma
BUY VISOMITIN SKQ1 5ML EYE DROPS / Mitochondria
https://www.ebay.com/p/10034911144
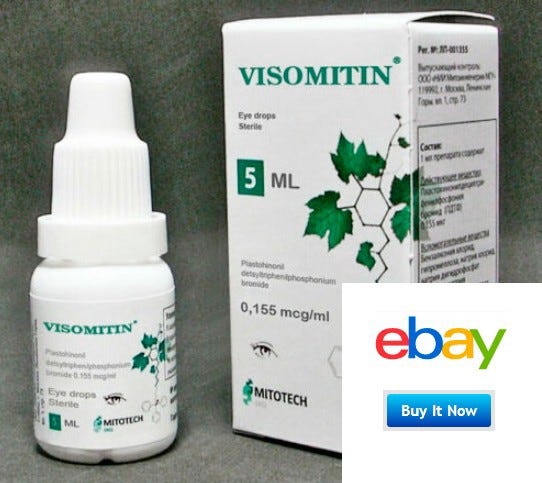
BUY VISOMITIN SKQ1 5ML EYE DROPS / Mitochondria
https://www.ebay.com/p/10034911144
Causes of degenerative changes in the anterior segment of the eye( POH), the role of oxidative stress. The main directions of therapy for degenerative changes in the POH. Antioxidant drugs in ophthalmology Classification of dry eye syndromes (SSS) Systemic diseases accompanied by the development of SSG, the role of the patient’s age. Degenerative changes in the tissues of the anterior part of the eye, secondary corneal dystrophy Clinical cases of the use of the mitochondrial antioxidant Visomitin in patients with corneal degeneration. Translated using translated using Yandex Translator from Russian.



The role of SKQ1 (Visomitin) in inflammation and wound healing of the ocular surface // Ophthalmology and Therapy volume
url: https://link.springer.com/article/10.1007/s40123-018-0158-2
ABSTRACT Introduction: SkQ1 (Visomitin) is a novel mitochondrial-targeted antioxidant that holds promise in the treatment of inflammation associated with ocular surface diseases such as dry eye disease (DED) and corneal wounds. However, the specific role of SkQ1 in ocular surface epithelial tissue has yet to be explicated. The goal of this study is to identify roles of SkQ1 in conjunctival inflammation and corneal wound healing.
Methods: To determine the role of SkQ1 in inflammation, human conjunctival epithelial (HCjE) cell cultures were sensitized with proinflammatory cytokine tumor necrosis factor alpha (TNF-a) and interleukin 1 beta (IL-1b) followed by treatments with SkQ1. The production of inflammatory biomarker prostaglandin E2 (PGE2) and cell viability were quantitatively evaluated. To determine the role of SkQ1 in wound healing, human corneal limbus epithelial (HCLE) cell cultures were streaked to create wounds. The wound closure times, ability to support single HCLE cell proliferation and changes of cell migration in the presence of SB203580, an inhibitor of p38 mitogen-activated protein kinase (MAPK), were further compared. Results: The HCjE and HCLE cultures showed no apparent cytotoxicity to SkQ1 in concentrations up to 250 nM (HCjE) or 2500 nM (HCLE). The HCLE cultures showed no toxicity to SkQ1 at all the SkQ1 concentrations tested. SkQ1 significantly suppressed PGE2 production of HCjE at concentrations\300 nM (24 h) and 50 nM (48 h), apparently being SkQ1 dose and treatment time dependent. The wound closure rates were increased by 4% in 4 h and by 9% after 8–12 h in the presence of 50 nM SkQ1. Furthermore, as little as 25 nM of SkQ1 significantly stimulated HCLE single-cell proliferation. Lastly, the SkQ1-stimulated wound healing was completely abolished by SB203580. Conclusion: Results of the current study demonstrate that SkQ1 exhibits an anti-inflammatory role and can be safely applied to ocular surface epithelium up to a concentration of 300 nM (181 ng/ml) for 24 h. SkQ1 also significantly enhances corneal epithelial wound healing, likely through enhancement of cell proliferation and migration. The data provide solid support for SkQ1 as a promising new therapeutic strategy for treatment of conjunctival inflammation as well as corneal wounds. Funding: This study was sponsored by Mitotech SA Pharmaceuticals.
Keywords: Conjunctiva; Cornea; Epithelial cell cultures; Inflammation; SKQ1; Wound healing
INTRODUCTION
Reactive oxygen species (ROS), oxidative stress and inflammation have been shown to be important in many eye diseases including dry eye disease (DED), atopic keratoconjuctivitis (AKC), conjunctivochalasis, age-related macular degeneration and glaucoma-related inflammation [1–9]. Oxidative stress is suggested to be a causative factor in the pathogenesis of DED [4] by driving the onset of inflammation and increasing angiogenesis. Recent progress in DED research acknowledges that ocular surface inflammation and damage play etiologic roles and that inflammation is the core mechanism in the pathogenesis of DED [10–14]. Thus, addressing the relationship among ROS production, lipid peroxidation-related membrane damage and the inflammatory process seen in DED may lead to an effective novel approach to DED treatment [4, 14]. Oxidative stress has also been confirmed to play important roles in corneal wound healing [15–17]. Wound healing is a recovery process of tissues or organs in response to trauma and injury, beginning with initial inflammatory responses at the wound loci by neutrophils, monocytes and macrophages followed by the formation of granulation tissue with fibroblasts and myofibroblasts and ending with vascularization and epithelization, where ROS has been shown to participate in all three phases [18, 19]. An unhealed wound is often a result of decreased ROS production and chronic inflammation [20]. A new drug capable of controlling ROS production may hold great promise to achieve perfect wound healing of the cornea. SkQ1 actively penetrates across the cellular membrane and accumulates in the inner leaflet of the mitochondrial membrane where it is reduced or recharged in a controlled manner. The transition between the two forms helps to reduce damages caused by mitochondrial ROS overproduction [22, 23]. Previous studies on tissue cultures and animal models indicate great therapeutic potentials of SkQ1 and its analogs in diseases with various inflammatory conditions, aging and wound healing [20, 21, 24–31]. Although SkQ1 has been formulated as an eye drop (Visomitin) in Russia and even underwent a small clinical trial for treatment of DED in the USA [32], very little is known about the role and mechanism of SkQ1 in inflammation or wound healing of the ocular surface. This study explored the roles of SkQ1 in conjunctival epithelial inflammation and corneal epithelial wound healing using in vitro human conjunctival and corneal epithelial cell culture models
METHODS
General Methodology This article does not contain any studies with human participants or animals performed by any of the authors.
Cells and Culturing Human conjunctival epithelial (HCjE) and corneal epithelial (HCLE) cells were cultured following the protocol provided with the frozen cell stock and consistent with previous publications [33, 34]. Growth and maintenance were under standard cell culture conditions (37C, 5% CO2 and 100% relative humidity) until confluent, following established protocols [34] (see Supplementary 1 for detailed methodology).
SkQ1 Stock and Working Solutions The stock solution of SkQ1, Mitotech-6, batch no. 040414M, was kindly provided by Mitotech S.A. Pharmaceuticals. It was prepared in a solvent containing sterilized 50% ethanol-0.9% NaCl solution. The SkQ1 concentration was 100 mg/ml. Following the instructions, this stock was stored at — 20 C until use; it can be stored for at least 1 year from the preparation date. The working solutions of SkQ1 were prepared as 2 9 serial dilutions in the maintenance medium as described above. Both the stock solution and working dilutions were kept in light-proof containers before and after being applied to the cultures.
Cell Viability, Dosing and Safety Ranges Cell Viability Determination with Varying Concentrations of SkQ1 and Diluent To evaluate the proper dosing and safety range of SkQ1 on healthy HCjE and HCLE cell cultures, the SkQ1 stock solution from Mitotech SA was serially diluted in culture medium, ranging from 0.25 nM to 250,000 nM, 10 9 range. As a control, the diluent (50% ethanol) was also serially diluted in culture medium, ranging from 0 to 0.75%, equivalent to the concentrations carrying over from the SkQ1 stock. Cellular morphology changes were observed and recorded by a NIKON D5000 digital camera installed on an Olympus CK2 Inverse Phase Contract Microscope and transferred to a linked computer that ran ‘‘Control My Nikon’’ v3.0 software for further analysis. Quantitative cell viability was determined by the 3-[4,5- dimethylthiazol-2-yl]-2,5 diphenyl tetrazolium bromide (MTT) assay protocols established in the laboratory [35]. The MTT assay is based on the conversion of MTT into formazan crystals by living cells, which determines mitochondrial activity optically [36]. The calculated cell viability values were plotted against the SkQ1 concentrations used (see Supplementary 2 for detailed MTT methodology).
Cell Viability Determination when SkQ1 Is Combined with Sensitizing Reagent IL-1b or TNF-a To rule out potential interferences from the combination of SkQ1 and pre-sensitizing agents on HCjE cells, the HCjE cell cultures were presensitized for 1 h with 10 ng/ml of TNF-a or IL1b as previously reported [37] and then exposed to various concentrations of SkQ1 in combination with the following: (1) culture medium only; (2) IL-1b (10 ng/ml); (3) TNF-a (10 ng/ml). Each treatment had three repeats. Controls included medium alone, TNF-a alone, IL-1b alone and SkQ1 alone. Exposure times under the standard incubation were 36 h. Experiments were run on 96-well plates, and results were photographically recorded.
Role of SkQ1 in Inflammation of HCjE Conjunctival Epithelial Cells SkQ1 Treatment of HCjE Inflammation The HCjE cells were grown in 96-well plates until reaching nearly 95% confluency, washed twice with pre-warmed medium and then given pre-treatments with freshly made maintenance medium containing varying concentrations of SkQ1 as indicated for 1 h. Then, 1 ll of freshly made IL-1b or TNF-a working solution was added to the SkQ1 pre-treated cells at final concentrations of 10 ng/ml. The treatments continued for 24 h. The control wells received the maintenance medium only. To acquire statistically meaningful results, each treatment in one experiment was repeated at least twice, and the entire experiment was repeated at least three times.
PGE2 Assay At 24- or 48-h SkQ1 treatments, 10 ll of samples was taken from each well and immediately analyzed for the concentrations of an inflammatory biomarker, prostaglandin E2 (PGE2), released in the culture medium. A monoclonal enzyme immunoassay (EIA) kit (Cayman Chemical Co., Ann Arbor, MI) was used following the manufacturer’s instructions and the protocol established in the laboratory [33]. The degrees of PGE2 production were then calculated and plotted using the formula described in the Methods section.
Role of SkQ1 in Corneal Wound Healing Wound Creation A single, one-dimensional ‘‘streak’’ defect in the epithelial cell lawn was created in a well by a common scratch method utilizing 200-ll or
1-ml Eppendorf pipette tips, referring to published methods [15, 38, 39]. SkQ1 Treatment Groups Immediately after a wound was created, the cultures were gently washed twice with the expired Keratinocyte Serum Free Medium (KSFM, Gibco) to remove remaining dead cell sheets or debris and then re-grown in the maintenance medium. The SkQ1-treated groups received 50 nM SkQ1 in the maintenance medium. The control groups received the medium only with the solvent (ethanol). Since in the preliminary experiments the medium containing ethanol at 0.000002% concentration, equivalent to the amount that would be carried over from a 50-nM SkQ1 working solution, was tested to be intoxicating to the cells, we omitted the addition of the vehicle solvent to the controls.
End Point Measurements of the Corneal Wound-healing Process To monitor and compare the wound-healing processes in the SkQ1-treated groups with those of the control groups, three markers were made alongside each wound at the bottom outside with a fine-tip marker pen to label the loci to be photographed immediately after scratching. These markers were used for alignment purposes: each was photographed together with the individual wounding spot at nearly the same orientation for all the photographing time points at 0, 4, 8 and 12 h from scratching till the wound was completely closed (healing). Photos were taken by a NIKON D5000 digital camera installed on an Olympus CK2 Inverse Phase Contract Microscope. Image files were recorded into a linked computer via ‘‘Control My Nikon v3.0’’ software.
Cell Proliferation To evaluate whether SkQ1 has a role in HCLE cell proliferation, extensively diluted single HCLE cells were inoculated into a 24-well plate in the growth and maintenance medium supplemented with various concentrations of SkQ1 ranging from 0 to 400 nM. Cell growth was tracked photographically at day — 1, 2 and 6 (corresponding to 24, 48 and 144 h). A standard MTT assay was conducted, except the stained cells were dried and photographed prior the addition of acidic isopropanol to dissolve formazan and to measure the absorbance at A572 and 690 nm. Two independent experiments were conducted, each with two repeats (see Supplementary 2 for detailed MTT methodology)
Cell Proliferation To evaluate whether SkQ1 has a role in HCLE cell proliferation, extensively diluted single HCLE cells were inoculated into a 24-well plate in the growth and maintenance medium supplemented with various concentrations of SkQ1 ranging from 0 to 400 nM. Cell growth was tracked photographically at day — 1, 2 and 6 (corresponding to 24, 48 and 144 h). A standard MTT assay was conducted, except the stained cells were dried and photographed prior the addition of acidic isopropanol to dissolve formazan and to measure the absorbance at A572 and 690 nm. Two independent experiments were conducted, each with two repeats (see Supplementary 2 for detailed MTT methodology)
Data Analysis Evaluation of Inflammation on HCjE The degree to which PGE2 was produced by HCjE cells sensitized by IL-1b or TNF-a under various concentrations of SkQ1 was compared with the corresponding degree of PGE2 production from cells without sensitization. Data were normalized for interpretation of results. Results were reported as mean ± SD (r). A Student’s t test was performed for statistical analysis (see Supplementary 3 for specific normalization calculations).
Evaluation of Wound Healing on HCLE The areas of wounds at various time points were calculated with ImageJ Fiji software. The whole wounded areas were used for the calculation against a reference with known dimensions, and rates of wound healing (%) of a specifically marked location at a particular time point were calculated. Results were reported as mean ± SD (r) (see Supplementary 3 for specific calculation).
Role of SkQ1 in conjunctival epithelial inflammation (24 h post-treatment). SkQ1 at concentrations \ 300 nM can effectively reduce the PGE2 production in HCjE as observed at 24 h of treatment. Data are the mean of four independent experiments, each with duplicate measurements
RESULTS Cell Viability, Dosing and Safety Ranges Cell Viability Determination with Varying Concentrations of SkQ1 and Diluent The cell viability did not significantly decrease until the SkQ1 concentration approached 250 nM (74% in HCjE) or 2500 nM (85% in HCLE), as quantitatively measured by MTT analysis. These results were confirmed on HCjE cells by morphologic observation under the microscope. With either method, the solvent alone (ethanol) did not show any apparent toxicity at any concentrations tested. The toxic concentration for 50% of cells (TC50) where SkQ1 reduces HCjE cell viability by 50% was calculated to be 317 nM (Fig. 1); we used 300 nM of SkQ1 for conjunctival inflammation experiments. Since SkQ1 at 2500 nM concentration, much higher than the range (0–400 nM) used for HCLE wound-healing experiments, only reduced HCLE cell viability by 15%, the TC50 for HCLE did not need to be calculated.
Results of four independent experiments are shown in Fig. 2. At the 24-h time point and compared with the untreated controls, the SkQ1-treated cells significantly reduced PGE2 production at concentrations from 25 to 175 nM. The strongest inhibitory effects appeared at 175 nM, apparently being SkQ1 dose dependent. When the SkQ1 concentrations were further increased, between 200 and 300 nM, a strong inhibitory effect was still shown, but the ratios were reduced, perhaps because of the cytotoxicity. After 48 h of SkQ1 treatment, the inhibition of PGE2 production was still noticeable, especially when SkQ1 concentrations were\50 nM, apparently being SkQ1 dose dependent (Fig. 3). At concentrations [ 50 nM, significant
Role of SkQ1 in HCLE Wound Healing SkQ1 in Wound Healing in HCLE Cell Cultures A representative pair of wound-healing results after streaking is shown in Fig. 4, in the presence or absence of 50 nM SkQ1. As shown in Table 1, the addition of 50 nM of SkQ1 significantly stimulated the wound-healing process in HCLE cell cultures: a 4% increase after 4 h of SkQ1 treatment (p = 0.015) and 9% increase after 8–12 h of SkQ1 treatment (p = 0.007 and 0.003, respectively). Role of SkQ1 in HCLE Cell Proliferation As shown in Fig. 5a, b, compared with the no SkQ1 controls, the addition of 25–200 nM of SKQ1 significantly stimulated the single cell proliferation in maintenance medium, as indicated by the numbers of the viable cells stained with MTT reagent. Promotion of proliferation was seen clearly yet less significantly when the growth medium was used. Role of SkQ1 in HCLE Cell Migration As shown in Fig. 6a, b, in the absence of SB 203580, the ratios of wounded areas between 0 and 10 h of treatment were 42% in the no SkQ1 groups and 25% in the 400 nM SkQ1-treated groups; the addition of 400 nM SkQ1 contributed a 17% increase in wound healing. However, when 10 lM of SB 203580 was present, the ratios of wound areas between 0 and 10 h were up to 70% in the no SkQ1 controls and 69% in the 400 nM SkQ1-treated groups, nearly completely abolishing the stimulating effect of SkQ1 on HCLE wound closure, with an
DISCUSSION In this study, we first demonstrated that SkQ1 can be safely applied to human conjunctival epithelial cells up to a concentration of 300 nM (181 ng/ml) to reduce PGE2 production (Fig. 1). PGE2 is well recognized as an inflammatory mediator that can be used for quantitative measuring of inflammatory responses of inflamed tissues or cells [37, 41]. Using this established cell culture model, we demonstrated that addition of SkQ1 significantly reduced the production of PGE2 in both TNF-a- and IL-1bsensitized HCjE cells after 24 h of SKQ1 treatment compared with no-SkQ1 control and in an apparently dose-dependent manner (Fig. 2). The inhibition extended up to 48 h in concentrations \ 50 nM, whereas higher concentrations appeared to cause cytotoxicity (Fig. 3). These results provide the first direct evidence that SkQ1 has an anti-inflammatory role in vitro on HCjE cells. We then demonstrated that SkQ1 significantly shortened the wound closure times of the human corneal epithelial cells (Fig. 4 and Table 1) at concentrations of 50 nM. Both the epithelial proliferation (Fig. 5) and migration (Fig. 6) were significantly enhanced. These results demonstrated that SkQ1 enhances corneal epithelial wound healing. Taken together, the results of the current study support that SkQ1 is a promising new therapy to inhibit inflammation of the human conjunctival epithelia and promote wound healing of the human corneal epithelia. The present study provides the first direct evidence that SkQ1 has anti-inflammatory effects in vitro on HCjE cells, and its anti-inflammatory role is likely through participating epithelial innate immunity. SKQ1 showed no apparent toxicity until the concentration approached 250 nM. This is consistent with previously published data that demonstrated a SkQ1 eye drop containing 250 nM SkQ1 could effectively prevent and reverse uveitis or even return vision to blind animals [42]. Our studies further demonstrated that increasing concentrations of SkQ1 [ 250 nM could cause cellular morphologic changes and cell death. The TC50 was determined to be 317 nM. At concentrations [ 300 nM (24 h) or 50 nM (48 h), SkQ1 displayed significant cytotoxicity. Therefore, the inhibitory effect on PGE2 production decreased and could not be accurately assessed. This indicates that SkQ1 \ 250 nM is biologically safe to human conjunctival epithelial cell cultures. Similarly, SkQ1 appeared not to be cytotoxic to HCLE cells at concentrations \ 2500 nm, indicating that SkQ1 at concentrations \ 250 nm is biologically safe for both corneal and conjunctival epithelial cells. The present study further demonstrated that stimulation of corneal wound healing by SkQ1 was nearly completely abolished in the presence of 10 lM SB203580 (Fig. 6). SB203580 is a p38 mitogen-activated protein kinase inhibitor that has been shown to completely suppress wound closure of the corneal epithelial cells [43], indicating the extracellular signal-regulated kinase (ERK) and p38 pathways of the mitogen-activated protein kinase cascade are involved in SkQ1-stimulated HCLE cell migration. It was also interesting to note that the promotion of HCLE cell migration appeared more effective in the presence of 400 nM of SkQ1 and SB 203580 (Fig. 6a) than 400 nM SkQ1 only (no shown), where the cytotoxicity of SkQ1 was clearly seen in the absence of the inhibitor. It is possible that SkQ1 indirectly counter-plays the inhibitory role of SB203580 in p38 kinase via a cellular molecular networking mechanism yet to be determined. It is also possible that SkQ1 accumulates in and has a greater effect on ‘‘younger’’ individual growing cells than ‘‘older’’ and less proliferating cell masses or sheets. SkQ1 appears to be more toxic in proliferating single cells than in the nearly confluent cell sheets. This may imply that the effect of SkQ1 on corneal epithelial wound healing can be affected at least by the cell growth stage as well as the density of the cultures. Results of the current study are based on human conjunctival and corneal epithelial cell lines. Further studies with primary human conjunctival and corneal cells and appropriate animal models are needed to establish the therapeutic values of SkQ1 in ocular inflammatory diseases. Mitochondrial ROS are well known in the regulation of apoptosis under both physiologic and pathologic conditions, especially when cells are inflamed [44]. It will be important to determine the role of SkQ1 in apoptosis of the ocular epithelium.
CONCLUSIONS Results presented in this study provide solid evidence to support SkQ1 as a promising new therapy for corneal trauma and wound damages. Given its in vitro efficacy in both conjunctival epithelial inflammation and corneal epithelial wound healing, SkQ1 may have a role as a new anti-inflammatory therapeutic modality in inflammatory conditions of the ocular surface such as chronic allergic conditions or DED. It may also have utility in the context of wound healing, where rapid closure of surface wounds reduces the risk of infection and other vision-threatening complications. Further investigation is warranted to explore the therapeutic potential and underlying mechanistic implications of SkQ1 on ocular surface inflammation and wound healing
Results of a Multicenter, Randomized, Double-Masked, Placebo-Controlled Clinical Study of the Efficacy and Safety of Visomitin Eye Drops in Patients with Dry Eye Syndrome
DOI 10.1007/s12325–015–0273–6
Vladimir V. Brzheskiy . Elena L. Efimova . Tatiana N. Vorontsova . Vladimir N. Alekseev . Olga G. Gusarevich . Ksenia N. Shaidurova . Alla A. Ryabtseva . Olga M. Andryukhina . Tatiana G. Kamenskikh . Elena S. Sumarokova . Eugeny S. Miljudin . Eugeny A. Egorov . Oleg I. Lebedev . Alexander V. Surov . Andrii R. Korol . Illia O. Nasinnyk . Pavel A. Bezditko . Olena P. Muzhychuk . Vladimir A. Vygodin . Elena V. Yani . Alla Y. Savchenko . Elena M. Karger . Oleg N. Fedorkin . Alexander N. Mironov . Victoria Ostapenko . Natalia A. Popeko . Vladimir P. Skulachev . Maxim V. Skulachev
ABSTRACT Introduction: This article presents the results of an international, multicenter, randomized, double-masked, placebo-controlled clinical study of Visomitin (Mitotech LLC, Moscow, Russian Federation) eye drops in patients with dry eye syndrome (DES). Visomitin is the first registered (in Russia) drug with a mitochondria-targeted antioxidant (SkQ1) as the active ingredient. Methods: In this multicenter (10 sites) study of 240 subjects with DES, study drug (Visomitin or placebo) was self-administered three times daily (TID) for 6 weeks, followed by a 6-week follow-up period. Seven in-office study visits occurred every 2 weeks during both the treatment and follow-up periods. Efficacy measures included Schirmer’s test, tear
break-up time, fluorescein staining, meniscus height, and visual acuity. Safety measures included adverse events, slit lamp biomicroscopy, tonometry, blood pressure, and heart rate. Tolerability was also evaluated. Results: This clinical study showed the effectiveness of Visomitin eye drops in the treatment of signs and symptoms of DES compared with placebo. The study showed that a 6-week course of TID topical instillation of Visomitin significantly improved the functional state of the cornea; Visomitin increased tear film stability and reduced corneal damage. Significant reduction of dry eye symptoms (such as dryness, burning, grittiness, and blurred vision) was also observed. Conclusion: Based on the results of this study, Visomitin is effective and safe for use in eye patients with DES for protection from corneal damage. Funding: Mitotech LLC. Keywords: Dry eye syndrome; Mitochondria-targeted antioxidants; Ophthalmology; SkQ1
INTRODUCTION Dry eye syndrome (DES) is a multifactorial disease of the tears and ocular surface that results in symptoms such as discomfort, visual disturbance, and tear film instability, with potential damage to the ocular surface. DES is accompanied by increased osmolarity of the tear film and inflammation of the ocular surface [1]. Increased osmolarity of the tear film can lead to morphological changes in the cornea and conjunctiva; activation of the inflammatory cascade can induce apoptotic cell death in the corneal epithelium and reduce the number of conjunctival goblet cells [2, 3]. Prevalence of DES increases with age, significantly affecting the quality of life of senior patients [1, 4, 5]. Artificial tear treatment that is typically used to alleviate DES symptoms does not target the cause of the disease. Therefore, there is a need for drugs with reparative action that help to protect against the onset and progression of corneal lesions associated with DES [5, 6]. One promising therapeutic approach is protection of the anterior segment of the eye from oxidative stress, as oxidative stress and reactive oxygen species (ROS) have been shown to play an important role in age-related eye diseases in general, and in DES in particular [7, 8]. One of the main sources of ROS in cells is mitochondria, which synthesize ATP and are the main consumers of molecular oxygen in the body [9, 10]. Natural antioxidants are not capable of penetrating mitochondria and neutralizing the excessive amounts of free radicals generated by these organelles. Only recently a new class of chemical compounds — mitochondria-targeted antioxidants — capable of neutralizing mitochondrial ROS has been developed [11, 12]. The active ingredient SkQ1 in Visomitin (Mitotech LLC, Moscow, Russian Federation) ophthalmic solution belongs to this class of compounds and is capable of targeting and neutralizing mitochondrial ROS [13, 14]. Pre-clinical studies have shown that SkQ1 is a promising agent for treating ophthalmic diseases associated with oxidative stress [15–17]. Visomitin is the first drug containing SkQ1 that has been studied in clinical trials. In an initial clinical study, Visomitin demonstrated efficacy in treating signs and symptoms of DES compared to an artificial tear treatment used as a control [18]. Subsequently, Visomitin ophthalmic solution was approved in Russia in December 2011 and has since been marketed there as a prescription product. The purpose of the second clinical study that is the subject of this article was to assess the safety and tolerability of Visomitin and to evaluate the treatment and post-treatment efficacy of Visomitin compared with placebo (with the same artificial tears formulation used as a control in the initial Visomitin study) in patients suffering from DES with mild to moderate severity levels.
METHODS A double-masked, randomized, placebo-controlled clinical trial of the efficacy and safety of Visomitin was conducted at 10 ophthalmic research centers in Russia and Ukraine. The study was conducted in accordance with good laboratory practice guidance, the Declaration of Helsinki, and Russian/Ukrainian regulatory requirements, and was registered with the Russian Ministry of Health. The study was approved by local ethics committees, and each subject was provided with a written informed consent to participate. Study Population The study included 240 patients (50 men and 190 women) aged 18–75 years demonstrating clinical signs of DES of mild to moderate severity levels, as determined by the investigator. Patients were excluded if they: had hypersensitivity reaction to the SkQ1 drug; had low visual acuity [VA; Sivtsev-Golovin scores [19] of \0.3 adjusted for worst seeing eye, with 1.0 = good vision (equivalent to 20/20 on the Snellen chart) and approaching 0.0 = poor vision (e.g., 0.1 = 20/ 200 on the Snellen chart)]; had elevated intraocular pressure (IOP; [21 mm Hg); had acute inflammatory diseases of the eye; or had other diseases or conditions (e.g., diabetes, drug or alcohol addiction, pregnancy, breast feeding) that could have had an impact on treatment performance evaluation or safety. There were no limitations for use of concomitant therapy during the study. Rare cases of ocular concomitant therapy usage are summarized in Table 1. Apart from the 11 patients mentioned in Table 1, no other ocular concurrent eye drops or other medications were used. Other concomitant therapy included a broad range of medications prescribed for non-ocular concomitant diseases. Study Design All subjects who met the inclusion and exclusion criteria were randomized into two groups of 120 patients each. In the treatment group, patients were assigned Visomitin eye drops, and in the control group, patients were given placebo (in a double-masked manner). Patients were instructed to instill one drop per eye three times a day for 6 weeks. Visomitin eye drops contained 0.155 lg/mL (250 nM) SkQ1. The placebo consisted of benzalkonium chloride (0.1 mg), hypromellose (2 mg), sodium chloride (9 mg), sodium dihydrogen phosphate dihydrate (1.14 mg), and sodium dihydrogen phosphate dodecahydrate (0.96 mg) per 1 mL, pH 6.5, and was identical to the treatment drug Visomitin formulation except that the active ingredient SkQ1 was absent. During the treatment period, patients were required to visit their research center for examination every 2 weeks. After the end of the treatment period, patients discontinued instillations and visited the center every 2 weeks for another 6 weeks (follow-up observation period).The study had 7 scheduled visits, with Visits 1–4 occurring during the 6 weeks of treatment, and Visits 5–7 occurring during the follow-up observation period (the next 6 weeks). At the visits, the following standard ophthalmological examinations were used to evaluate the effectiveness of the therapy: Schirmer’s test, tear break-up time (TBUT) test, fluorescein staining, meniscus height, and VA. In addition, to evaluate safety, slit lamp biomicroscopy was conducted to assess pathological changes, tonometry was conducted to determine IOP, blood pressure and heart rate were measured, concomitant therapy drugs were noted, and complaints and adverse events (AEs) were recorded. After completion of treatment, patients and physicians were asked to evaluate the efficacy, tolerability, and residual efficacy (continued drug effect after treatment is terminated) using a visual analog scale (VAS) from 0 to 100 points, where 0 = no effect and 100 = very good effect. Efficacy measurements for both eyes for each patient were pooled by group (treatment group or placebo group) to develop group statistics. For these parameters, positive response to treatment was assessed (i.e., number of eyes that showed an improvement by more than 5% from the initial level).
Study Procedures All criteria for evaluating the results of efficacy procedures were determined prospectively. The Schirmer’s test was performed by applying certified test strips in the lower eyelid of each eye for 5 min without anesthesia. Schirmer’s test results for tear production were evaluated as follows [5, 6]: wet segment of C25 mm = hyperlacrimation, 15–24 mm = normal, 10–14 mm = mild level of tear production dysfunction, 5–9 mm = moderate, \5 mm = severe. The TBUT test for TBUT was performed in accordance with standard procedures. Results of the TBUT samples were evaluated as follows: 10 s or more = normal [20], 5–9 s = mild level of TBUT dysfunction, 3–4 s = moderate, \3 s = severe [5, 6]. Corneal staining was performed using fluorescein strips, with the cornea divided into five zones: central, superior, inferior, nasal, and temporal. The number and size of corneal lesions were evaluated separately for each zone using the following scale: no staining = 0 points, 1–15 punctate staining areas = 1 point, [15 areas of punctate staining or one continuous area = 2 points, [15 areas of punctate staining and one or more continuous area = 3 points. The total damage to the cornea was determined by adding the scores for all five zones. Tear meniscus height was determined before other procedures were performed. VA was determined using Sivtsev–Golovin tables illuminated at 600–1000 lux at a 5 m distance. Statistical Analysis Two-sided levels of significance and confidence intervals were calculated and reported, and a statistical level of significance of 0.05 was used (unless otherwise indicated). For efficacy parameters, significance assessments were performed using a modified t test (with Fischer arcsin-frequency conversion for frequency variables), v2 test with Mantel–Haenszel correction, and Fisher’s exact test. Statistical analysis was performed using SAS software version 6.12 (SAS Institute Inc., Cary, NC, USA). No changes were made to the methodology after the trial began. One post-study subgroup analysis was conducted for baseline total corneal damage (elevated or not elevated), as discussed in this article. No other subgroup analyses were conducted, and no interim or other adjusted analyses were conducted.
RESULTS Subject Demographics and Disposition The Visomitin treatment group and the placebo group were statistically comparable in terms of gender and age: both groups were dominated by female patients, and average age in the placebo group was 46 years and in the treatment group was 47 years (Table 2). All randomized subjects received treatment. In addition to the 235 patients who completed the study (116 in the placebo group and 119 in the Visomitin treatment group), 3 patients assigned to the placebo group withdrew consent to participate in the study for personal reasons, 1 patient assigned to the placebo group dropped out due to an AE of allergic conjunctivitis, and 1 patient assigned to the Visomitin group dropped out as a result of a serious AE (SAE; see ‘‘Safety Results’’).
Safety Results AEs were typical of those reported by patients with DES and included burning sensation after
instillation of the test drug, which is commonly reported after use of artificial tear formulations in the conjunctival cavity [5, 6]. One SAE, a fatal myocardial infarction that was considered unrelated to drug treatment, was reported in the Visomitin group during the follow-up observational period. In addition, 55 AEs were reported: 21 AEs in the placebo group and 34 AEs in the Visomitin group. AEs considered definitely related to the study drug, in addition to burning after instillation (which occurred in similar numbers in both treatment groups), included eye redness after instillation and scratchiness in the Visomitin group, and blurring vision after instillation, and itchiness, redness, and hypersensitivity reaction including papular eruption on the hands, neck, and chest in the placebo group. Other AEs that were considered probably or possibly related to drug treatment included lacrimation or tingling on instillation, eye dryness, and itchiness in the Visomitin group, and prominent eyelid and conjunctiva hyperemia, swelling similar to allergy reaction, and film-like coating, tingling, lacrimation, or scratchiness after instillation in the placebo group. AEs classified by the degree of relationship to the drug are shown in Table 3. One AE of allergic reaction that led to discontinuation of treatment was reported (in the placebo group). No other AEs resulted in discontinuation of treatment. There were no AEs of elevated IOP, blood pressure, or heart rate. Tolerability test results using a tolerability VAS showed that Visomitin was well tolerated: the average tolerability rating on a scale from 0 to 100% (with 0 = absolutely intolerable and 100 = very well tolerated) was approximately 90%. Based on the safety results, Visomitin was categorized
as safe for use in patients with DES and well tolerated. Visual Acuity Patients had relatively high VA levels at the time of enrollment prior to treatment (average uncorrected Sivtsev–Golovin VA score was 0.63 ± 0.02 in the placebo group and 0.62 ± 0.02 in the Visomitin group; average corrected VA was close to 1 in both groups). VA statistically significantly (P\0.05) improved over the 6 weeks of therapy in both groups. At Visit 4, the average uncorrected VA was 0.64 ± 0.02 in the placebo group and 0.63 ± 0.02 in the Visomitin group, with no statistically significant difference between the groups. This VA improvement during the treatment period was probably due to the effect of hypromellose present in both the Visomitin and placebo formulations. Meniscus Height Meniscus height at the time of enrollment was 256.3 ± 9.3 lm in the placebo group and 259.8 ± 8.1 lm in the Visomitin group. At Visit 4, meniscus height statistically significantly (P\0.001) improved in both groups (308.3 ± 9.8 lm in the placebo group and 298.1 ± 8.4 lm in the Visomitin group). At Visit 7, meniscus height scores worsened in both groups (262.6 ± 7.8 lm in the placebo group and 274.0 ± 6.9 lm in the Visomitin group), with no statistically significant difference between the groups. Schirmer’s Test Schirmer’s test scores at baseline averaged 11.1 ± 0.5 mm in the placebo group and 11.7 ± 0.6 mm in the Visomitin group, which corresponds to a mild level of tear production dysfunction. At baseline, several patients suffered from hyperlacrimation, most patients showed a mild level of tear production dysfunction, a third of the population suffered from a moderate level of tear dysfunction, and about 10% were assigned to the ‘severe’ category according to the Schirmer’s test. By the end of the treatment (Visit 4), the average Schirmer’s test scores in both groups improved (increased) significantly (P\0.001) to 13.7 ± 0.4 mm in the placebo group and 14.2 ± 0.5 mm in the Visomitin group. At the end of the observation period (Visit 7), scores decreased almost to baseline levels, averaging 11.6 ± 0.4 mm in the placebo group and 12.2 ± 0.5 mm in the Visomitin group, with the difference between the groups not significant. These data suggest that the effect of Visomitin on tear production was positive, but did not dominate the effect of hypromellose over the 6-week treatment period.
TBUT Test The TBUT test is an important metric for DES severity as it reflects the overall tear quality throughout all layers of the tear film. At baseline, patients in both the Visomitin and placebo groups were predominantly assigned to the mild category of tear production dysfunction. TBUT test averages were 7.2 ± 0.2 s in the placebo group and 6.8 ± 0.2 s in the Visomitin group. Change in TBUT test results from baseline throughout the study visits is shown in Fig. 1. By the end of the treatment period (Visit 4), TBUT in patients treated with Visomitin increased (improved) slightly more than in patients in the placebo group (change from baseline was 2.9 ± 0.3 s in the placebo group and 3.1 ± 0.3 s in the Visomitin group, although these differences were not statistically significant). This effect persisted for at least 2 weeks after drug instillations were stopped. Some level of improvement of TBUT was maintained in patients in the Visomitin group even at the end of the follow-up observation period (Visit 7). Evaluation of Corneal Damage In this study, the fluorescein staining technique was used to evaluate the area of damage in five different zones of the cornea (central, superior, inferior, nasal, and temporal) and in the cornea overall (total damage score, as a sum of zone scores). The maximum possible total damage score was 15, and a total score of 3 or higher was considered abnormal. The damage level in the central zone of the cornea is one of the most interesting indicators in DES patients, as this zone is most prone to the influence of environmental factors. Figure 2 shows the average staining scores for the central zone of the cornea, and Fig. 3 shows the average total staining scores. Both central corneal zone damage and total corneal damage were significantly (P\0.001) reduced during the treatment period in both the Visomitin and placebo groups. During the follow-up observation period, a significant post-treatment effect was seen for Visomitin compared to placebo. The difference between the groups for central corneal fluorescein staining was statistically significant 2 weeks after treatment was stopped, at Visit 5 (P\0.01) and at Visit 6 (4 weeks post-treatment, P\0.05; Fig. 2). For total corneal fluorescein staining, the difference between the groups was statistically significant at Visits 5 and 6 (P\0.01 at both time points; Efficacy in Patients with Elevated Corneal Damage For an additional post-study analysis, all patient eyes were assigned to two subgroups according to the degree of their baseline total corneal damage: mild damage (below 3 points), and elevated damage (3 points or more). Sixty-five eyes treated with placebo and 57 eyes treated with Visomitin were assigned to the elevated corneal damage subgroup, which demonstrated the most pronounced effect of Visomitin. Average baseline Schirmer’s test scores for the ‘elevated corneal damage’ subgroup were 10.1 ± 1.0 mm for patients treated with placebo and 12.4 ± 1.2 mm for patients treated with Visomitin. At the end of the treatment period, Schirmer’s test score averages increased (improved) to 13.2 ± 0.9 mm in the placebo group and 14.6 ± 0.6 mm in the Visomitin group. After another 6 weeks of the follow-up observation period, Schirmer’s test scores returned to approximately baseline values in both groups. Differences between the placebo and Visomitin groups were not significant at any of the visits for the elevated corneal damage subgroup. However, positive response to treatment as reflected by an increase in Schirmer’s test scores by the end of the treatment period was 72% for placebo patients and 86% for Visomitin patients (P\0.1). Figure 4 shows the change from baseline in TBUT test results in patients with elevated corneal damage as a percentage. In this subgroup, for patients treated with Visomitin, average TBUT test results increased (improved) by 5 s by the end of treatment, compared to 4 s for patients receiving placebo. By the end of the follow-up observation period, the TBUT test score average for the Visomitin patients in this subgroup was 3 s higher than it was prior to treatment, compared to 1.5 s for patients receiving placebo. Corneal fluorescein staining results were notable for the subgroup of patients with elevated corneal damage. Figure 5 shows average central corneal staining scores in the elevated corneal damage subgroup, and Fig. 6 shows average total corneal staining in this subgroup. Corneal staining (corneal damage) was significantly reduced in patients treated with Visomitin compared to patients receiving placebo after 6 weeks of treatment, and this effect remained for the next 6 weeks of follow-up observation. Response to treatment (positive response = number of eyes with [5% improvement from baseline) for central corneal staining at the end of treatment (Visit 4) was 54% in placebo patients and 72% in Visomitin patients (P\0.05). Similar trends were observed in other areas of the cornea. Thus, corneal staining results for Visomitin showed significant therapeutic effect in protecting the cornea from damage in the general population of DES patients when compared to patients who received placebo, and this therapeutic effect was especially pronounced in the elevated corneal damage subgroup. Evaluation of Symptom Scores All symptoms were evaluated using a scale reflecting the frequency of symptoms: the greater the frequency of the symptom, the higher the score. Symptom changes (relative to the baseline visit) for dryness, grittiness, blurred vision, and worst symptom (estimated as the symptom with the highest score at baseline) are shown in Fig. 7 (for all patients) and Fig. 8 (for the elevated corneal damage subgroup). Statistical analysis indicated that blurred vision, dryness, grittiness, burning, and eye fatigue decreased during treatment in both the Visomitin and placebo groups, but the decrease in the Visomitin group was more pronounced, especially during the follow-up observation period. The differences between the groups were statistically significant for blurred vision 4 weeks after treatment was stopped (Visit 6, P\0.05) and 6 weeks post-treatment (Visit 7, P\0.01); for dryness (P\0.1) at Visits 6 and 7 (6 weeks post-treatment); for grittiness (P\0.1) at Visit 7; for worst symptom (P\0.01) at Visit 5, and at Visits 6 and 7 (P\0.001). This decrease was even greater for the elevated corneal damage subgroup (Fig. 8), with statistically significant differences between the groups for dryness 4 weeks after treatment was stopped (Visit 6, P\0.1); for grittiness after 6 weeks of therapy (Visit 4, P\0.05) and 2 and 4 weeks post-treatment (Visits 5 and 6, P\0.01 and P\0.05, respectively); and for blurred vision during treatment at Visit 2 (P\0.01), Visit 3 (P\0.05), and Visit 4 (P\0.01), and post-treatment at Visits 5, 6, and 7 (P\0.001). Worst symptom score also showed superior efficacy of Visomitin compared to the placebo group during the follow-up observation period, with differences between the groups during treatment at Visit 3 (P\0.1) and Visit 4 (P\0.05) and post-treatment at Visits 5, 6, and 7 (P\0.01). DISCUSSION The results of recent studies indicate that clinical and biological signs of DES often do not match the severity of symptoms [1, 22, 23]. Despite the large number of diagnostic techniques, there is no single ‘gold standard’ that can determine the severity of the syndrome and evaluate therapy effectiveness. For example, the commonly used Schirmer’s test is not sufficient to uniformly assess severity of DES. Basal tear deficit can be compensated by reflex tear production, in which case the Schirmer’s test result will not indicate decline and sometimes will even show an increase. Another standard method for diagnosing DES — corneal fluorescein staining — also has its drawbacks. Elevated levels of staining sometimes occur in patients who do not have DES [24]. Moreover, reproducibility of corneal and conjunctival staining has been shown to be rather low [25]. A study that involved 300 patients found tear osmolarity to be the best marker of DES severity [26]. However, conjunctival and corneal stainings were shown to have the highest correlation with DES severity among commonly used diagnosis techniques. According to ophthalmologists of the ODISSEY European Consensus Group, which studied 14 commonly used DES diagnosis techniques, a joint analysis of symptom scores and fluorescein staining scores is usually sufficient for assessing DES severity [21]. Taking the above DES assessment methods into consideration, a variety of measurement techniques was used in the randomized, double-masked, placebo-controlled clinical trial of Visomitin. Symptoms scores, slit lamp exam data, Schirmer’s test data, TBUT, and corneal fluorescein staining data were assessed in patients with mild to moderate DES levels during the course of this study. The study results of corneal fluorescein staining (Figs. 2 and 3) are of particular interest. It was demonstrated that the Visomitin group had significantly less corneal damage in the central zone after the sixth week of treatment than the placebo group. Furthermore, this effect persisted for at least 4 weeks after the therapy was terminated. This statistically significant difference between the efficacy of Visomitin and placebo was mainly driven by the subgroup of patients with more elevated corneal damage (with total fluorescein staining scores C3 at baseline). Based on Sullivan et al. [26], these patients can be described as a subgroup with a moderate degree of dry eye. Interestingly, TBUT values in this subgroup were significantly higher (improved) in patients treated with Visomitin than in patients treated with placebo at the end of treatment and for the duration of the follow-up observation period (Fig. 4). Results also showed improvement in the symptom of blurred vision in this subgroup (Fig. 7b). Tutt et al. [27] argue that blurred vision, typical for patients with dry eye, is associated with a decrease of tear film stability and, therefore, should correlate with TBUT measurements. The results of this study showed that SkQ1 eye drops did not affect tear production levels: Schirmer’s test scores increased in both the Visomitin and placebo groups and did not differ significantly between the groups. According to Lemp [28], results of the Schirmer’s test may significantly vary even throughout a single visit. Also, the Schirmer’s test appears to be more reproducible in patients with low test values, that is, more severe disease. Therefore, it is believed that the applicability of the Schirmer’s test in patients with mild to moderate dry eye is limited. It is known that inflammation plays an important role in the pathogenesis of DES [28]. An increase in soluble mediators (cytokines and proteases) in the tear fluid, adhesion molecule expression by the conjunctival epithelium, and T cell infiltration of the conjunctiva have all been observed in dry eye patients [29]. It was previously shown that the anti-inflammatory effect of SkQ1, which inhibits the NF-kB signaling pathway, can lead to a decrease in the level of expression of adhesion molecules and in leukocyte infiltration [30]. It is probable that the anti-inflammatory effect of SkQ1 plays an important role in reducing corneal damage in patients with DES. These data indicate that Visomitin not only has a moistening effect due to the presence of hypromellose, but also that the active compound SkQ1 restores corneal cell function and tear film stability, thus directly addressing the causes of DES. Improvements in both symptoms and corneal staining associated with DES are attributable to Visomitin treatment, suggesting a beneficial therapeutic effect of the drug. Furthermore, post-therapy efficacy of Visomitin for TBUT, corneal staining, and symptoms over 4 weeks of the follow-up observation period, especially in patients with moderate dry eye, shows a clear advantage of Visomitin over artificial tear treatments. One possible limitation of the study was that the severity of dry eye was determined by the investigator and not quantified or specified earlier in the study as to how the investigators would decide the subject’s dry eye was mild or moderate in severity. However, the better results in the higher corneal staining subgroup suggest that the population was mixed; also, no standardized definition or selection criteria of DES severity currently exists. Also, there are limitations of some of the dry eye tests themselves (e.g., Schirmer’s test, corneal fluorescein staining), as mentioned above; using several dry eye tests compensates somewhat for the test limitations. In addition, seasonality may contribute to a reduction in the effects of dry eye treatments as the drier season progresses. Also, environmental factors, such as subjects’ increased use of computers or reading or exposure to tobacco smoke or wind, may also decrease the effects of drug treatments. CONCLUSIONS Visomitin has several important benefits for dry eye patients. Visomitin has been shown to be safe and well tolerated in patients with DES. Visomitin exhibited a more pronounced and stable post-therapy effect in patients with DES than placebo. Visomitin is highly effective in protecting patients with DES from corneal damage. ACKNOWLEDGMENTS This study was funded by Mitotech LLC. The article processing charges and open access fee for this publication were funded by Mitotech S.A. Editorial services were provided by Linda Stein, Ora, Inc. Editorial services were funded by Mitotech S.A. All named authors meet the International Committee of Medical Journal Editors (ICMJE) criteria for authorship for this manuscript, take responsibility for the integrity of the work as a whole, and have given final approval for the version to be published. Disclosures. All authors participated in conducting this study and received compensation for their work. M. Skulachev is the general director of Mitotech LLC, the company that funded this study and markets Visomitin in Russia. V. Brzheskiy, E. Efimova, T. Vorontsova, V. Alekseev, O. Gusarevich, K. Shaidurova, A. Ryabtseva, O. Andryukhina, T. Kamenskikh, E. Sumarokova, E. Miljudin, E. Egorov, O. Lebedev, A. Surov, A. Korol, I. Nasinnyk, P. Bezditko, O. Muzhychuk, V. Vygodin, E. Yani, A. Savchenko, E. Karger, O. Fedorkin, A. Mironov, V. Ostapenko, N. Popeko, and V. Skulachev have nothing further to disclose.
Preventive and therapeutic effects of SkQ1-containing Visomitin eye drops against light-induced retinal degeneration
Abstract — The human retina is constantly affected by light of varying intensity, this being especially true for photoreceptor cells and retinal pigment epithelium. Traditionally, photoinduced damages of the retina are induced by visible light of high intensity in albino rats using the LIRD (lightinduced retinal degeneration) model. This model allows study of pathological processes in the retina and the search for retinoprotectors preventing retinal photodamage. In addition, the etiology and mechanisms of retina damage in the LIRD model have much in common with the mechanisms of the development of age related retinal disorders, in particular, with agerelated macular degeneration (AMD). We have studied preventive and ther apeutic effects of Visomitin eye drops (based on the mitochondriatargeted antioxidant SkQ1) on albino rat retinas dam aged by bright light. In the first series of experiments, rats receiving Visomitin for two weeks prior to illumination demon strated significantly less expressed atrophic and degenerative changes in the retina compared to animals receiving similar drops with no SkQ1. In the second series, the illuminated rats were treated for two weeks with Visomitin or similar drops without SkQ1. The damaged retinas of the experimental animals were repaired much more effectively than those of the con trol animals. Therefore, we conclude that Visomitin SkQ1containing eye drops have pronounced preventive and therapeu tic effects on the photodamaged retina and might be recommended as a photoprotector and a pharmaceutical preparation for the treatment of AMD in combination with conventional medicines.
url: https://link.springer.com/article/10.1134/S0006297914100113
DOI: 10.1134/S0006297914100113
Key words: retina, photoreceptors, photodamage, SkQ1, Visomitin
Reactive oxygen species (ROS), which are mainly generated by mitochondrial electron transport [13], can cause considerable damage to various cell types. This is especially true for the retina, which is exposed to photon flux during the entire life of an organism, and in addition its cells are characterized by high rate of oxygen metabo lism and high content of polyunsaturated fatty acids [4, 5]. Thus, conditions are created for regular production of a large amount of ROS that damage retinal structures, especially photoreceptor cells — the rods and cones [6 11]. It has been demonstrated on many in vitro and in vivo models that the penetrating cation 10(6′plasto quinonyl)decyltriphenylphosphonium (SkQ1) has high antioxidant activity due to its ability to remove ROS from the mitochondrial matrix [1214]. According to our earlier data [15, 16], the antioxi dant SkQ1 has protective effect on the neural retina, reti nal pigment epithelium (RPE), and choroid cultivated in preparations of the posterior segment of albino rat eyes, significantly reducing cell death in these structures and preventing macrophage transformation of RPE cells. In the present study we examined the ability of Visomitin SkQ1containing eye drops to provide prophylactic and therapeutic effects on albino rat retina damaged by expo sure to high intensity light according to the LIRD (light induced retinal degeneration) model [17, 18]. MATERIALS AND METHODS Visomitin eye drops were obtained from the Institute of Mitoengineering, Lomonosov Moscow State University. The composition of the eye drops (per 100 ml): 0.0155 mg SkQ1, 10 mg benzalkonium chloride, 200 mg oxypropyl methylcellulose, 900 mg sodium chlo ride, 810 mg sodium dihydrophosphate, 1.1635 g sodium hydrogen phosphate dodecahydrate, and water to total volume 100 ml. Animals. Healthy twomonthold female albino Wistar rats (n = 36) were used for the experiments, six of them serving as controls. Prior to the experiment, the ani mals were kept under normal vivarium conditions (12 h light/12 h dark); we conducted regular external inspec tion of their eyes. Upon completion of the experiment, the rats were decapitated under chloral hydrate (Sigma, USA) anesthesia (500 mg/kg intraperitoneally). All the procedures were consistent with the protocols approved by the Russian Academy of Sciences Commission on Bioethics and performed in compliance with the ethical rules of the European Directive FELASA2010. Damaging of albino rat retina using the LIRD model. Before illumination, the rats were kept in the dark for 14 h for dark adaptation of their eyes, and then they were exposed to bright light for 14 h. The highintensity visible light source was an NCDE 70W/DW RX7s metal halide lamp (NARVA, Germany) with the following specifica tions: power 70 W, luminous flux 50005500 lumens, color temperature 4000 K. The lamp was placed 2 m from the animal cages, which corresponded to intensity of reti na illumination of 25003000 lx. Preventive effect of Visomitin SkQ1containing eye drops on albino rat retina. Rats (n = 18) of the experi mental (n = 9) and control (n = 9) groups received, respectively, SkQ1containing eye drops or similar drops without SkQ1 (placebo) for 15 days; in both cases, one drop per day was placed in the left and right eyes of each animal. Upon completion of the course, the animals were illuminated with the light of high intensity, and then were kept in the dark for 3 h. Immediately thereafter, six rats from the experimental and six rats from the control groups were euthanized. The remaining animals (three from each group) were kept for 15 days under the usual conditions (without illumination or administration of the eye drops) and then were euthanized. Therapeutic effect of Visomitin SkQ1containing eye drops on albino rat retina. The rats (n = 12) were illumi nated with the highintensity light and then kept for 15 days under the usual light conditions. The experimental group (n = 6) of illuminated animals received SkQ1con taining eye drops, and the control group (n = 6) received drops of the same composition without SkQ1 (placebo); in both cases the animals received one drop per day in the left and right eyes. Upon completion of the 15days course of administration of the eye drops, the animals were euthanized. Histological processing. Eyeballs isolated immedi ately after decapitation of the animals were placed in a Bouin’s fixative solution, and after two days the posterior segment containing retina, choroid, and sclera was isolat ed. Fixed preparations of the eye posterior segment were then washed in 70% alcohol and were subjected to routine histological processing including dehydration in alcohols of increasing concentration and embedding the properly oriented samples in paraffin. Sagittal serial sections (500 from each eye, 7μm thick each) were obtained from the paraffin blocks using a microtome (Reichert, Austria). The sections fixed on slides (MenzelGlaser, Germany) were deparaffinized, rehydrated, stained with Carazzi’s hematoxylin and eosin following the standard protocol, and mounted in Canada balsam. Morphometric analysis. Serial histological prepara tions were studied using Vanox AH3 (Olympus, Japan), Jenaval (Carl Zeiss, Germany), and AxioScope A.1 (Carl Zeiss) microscopes. Microphotographs were obtained using an AxioCam MRc5 highresolution digital camera (Carl Zeiss). Viewing and processing of the micropho tographs, addition of scales, and morphometric measure ments were performed using the AxioVision 8.0 (Carl Zeiss) and Adobe Photoshop CS6 Extended (Adobe Systems, USA) softwares. The width of the outer nuclear retinal layer (ONL) was examined 3 h after photodamaging. To estimate the change in this parameter caused by the intense light, two retinal preparations were randomly chosen from each group of rats euthanized 3 h after photodamaging. Five hundred measurements of ONL width were carried out in 60 serial sections from each such preparation, in eight dif ferent areas from the dorsal to the ventral sides of the eye (two dorsoperipheral areas, two dorsocentral areas, two ventrocentral areas, and two ventroperipheral areas). ONL atrophic area size was evaluated 15 days after the highintensity illumination. For this, two retinal preparations with the most severe damage were selected from each group of rats euthanized 15 days after illumi nation. ONL atrophic area size was determined as the product of the number of consecutive sections with detected ONL atrophy, section width (7 μm) and the length of the atrophy zone (in μm) in each section. The length of the inner segments of photoreceptor cells was determined in the area of the highest lightsen sitivity on 60 sections randomly selected from each group. The data were processed to determine the statistically sig nificant differences of sample mean values using the Mann–Whitney test at p = 0.05. Results were calculated and plotted using the MS Office Excel and ORIGIN 8.1 programs RESULTS Retinal damage caused by bright light. The structure of the retina of intact albino rats (n = 6) was typical for healthy tissue [19]. Figure 1 shows that the neural retina (following the direction from its scleral to vitreal parts) consists of three nuclear layers containing neuronal cell bodies that are interleaved with two plexiform layers con sisting of neuronal processes. The layers interacting with RPE are located closer to the sclera; they include the outer segments and bodies of photoreceptor cells that together form the outer retina. The outer plexiform layer, inner nuclear layer (INL) formed of interneuron bodies, and ganglion cell layer are located more vitreally. The inner plexiform layer is located between INL and gan glion cell layer, which together form the inner retina. Damages typical for the LIRD models [17, 18, 20 26] were found in the retina of albino rats exposed to illu mination with bright light (n = 9). Usually, morphologi cal manifestations of early photoinduced damages typical for this model are found in the outer retina 24 h after illumination. They include destruction of the photore ceptor layer, the initial stage of ONL thinning, pyknosis of photoreceptor nuclei, as well as vacuolization, migra tion, and phagocytic activity of RPE cells [17, 27, 28]. In our case, shortening and disorganization of outer and inner segments of photoreceptor cells and pyknosis of their nuclei were detected 3 h after photodamaging. Such changes in photoreceptor chromatin indicating the beginning of apoptosis caused the decrease in ONL thick ness, thinning of the outer plexiform retinal layer formed by photoreceptor axons, and shifting of the bodies of pho toreceptor cells in the scleral direction. In addition, some RPE cells migrated in the vitreal direction (Fig. 1) and phagocytized the damaged segments of photoreceptor cells (not shown). In some cases the neural retina was peeled off the RPE and choroid, demonstrating bucklings (not shown) due to accumulation of edema fluid and cel lular debris underneath. All the observed damages of the outer retina were of multifocal nature, which indicates unequal photosensi tivity of its different areas. ONL thinning detected 3 h after photodamaging showed that dorsocentral retinal area was most susceptible (Fig. 2). Relatively late photoinduced damages typical for the LIRD model are manifested in albino rat retinas several days to several weeks after exposure to bright light. These changes are present in outer retinal layers in the form of subtotal ONL atrophy due to apoptotic death of photore ceptors and in inner retinal layers. In the latter case, it is manifested in the formation of microneuromas and chan nels and shifting of INL neurons in the scleral direction, resulting in significant general restructuring of the retina [17, 18, 2024, 26]. In our experiments, 15 days after the illumination all the animals developed pronounced atroph ic changes in ONL and retinal plexiform layers as well as accompanying migration of ONL and INL cells in the vit real and scleral directions, respectively (Fig. 3). Atrophic loci were also observed in the RPE layer (not shown). In addition, compensatory processes involving pri marily the Müller glia of the neural retina developed in the albino rat retina during 15 days after its illumination with bright light. For example, in retinal areas adjacent to the areas of ONL atrophy, hypertrophy of glial cells processes could be observed that was manifested in increase in their thickness and number. Cysts and chan nels for draining the edema fluid were developing mainly in the INL. Microneuromas were detected in the INL — the clusters of neuronalphenotype cells and randomly arranged bundles of their processes (Fig. 3). Complete destruction of retinal cytoarchitectonics up to indistin guishability of its layers could be observed in the most severely affected retinal areas. Photodamageassociated changes of the ganglion cell layer (GCL) were less expressed, but death of cells of this layer was observed in about one third of the retinal samples obtained from the illuminated animals. All the detected late retinal changes were of multifocal character; damaged areas in the inner retina usually accom panied the atrophy and other damages in the outer retina. Preventive effect of Visomitin SkQ1containing eye drops on retina of albino rats subjected to illumination with bright light. In this series of the experiments, albino rats received Visomitin SkQ1containing drops (experimental group) or similar drops without SkQ1 (control group) for two weeks prior to the illumination. The state of retina in the experimental and control groups was compared after 3 h and 15 days to determine whether Visomitin had a preventive effect on the retina of illuminated animals. The severity of early and late pathological processes in the retina, typical for LIRD models, served as the criteria for this comparison. Death of photoreceptors whose bodies are located in the ONL, resulting in the reduction of this layer thickness, is one of the earliest events of lightinduced retinal damage. Comparison of ONL thickness in different areas of the reti nal preparations (n = 2) obtained from animals of experi mental and control groups, as well as from the intact rats, showed that ONL thickness was significantly greater in the experimental group (animals receiving Visomitin) than in the control group 3 h after illumination (Fig. 2), the effect of Visomitin being most pronounced in the central area of the retina. The degree of preservation of the outer and inner segments of photoreceptor cells, evaluated based on their size 3 h after illumination, was shown to be greater in rats from the experimental group both in peripheral and central retinal areas (not shown). Finally, Visomitin was shown to cause 3fold reduction in the number of atrophic and dystrophic RPE loci adjacent to the areas of ONL damage and photoreceptor cell death (when compared to the control, Table 1). In general, according to quantitative assessment of the state of retina in the control and experi mental samples of the posterior eye segment, the results of which are presented in Table 1, 3 h after illumination the state of retina remained close to intact in the vast majority of animals of the experimental group. Fifteen days after illumination, ONL multifocal atrophy was quite pronounced, and so we compared atro phy in the areas of this layer in experimental and control retinal samples using two samples from each group of rats. The average area size of ONL atrophy in the experimen tal group of animals was found to be significantly smaller than in the control (Fig. 4), i.e. late changes in the outer neural retina were also sensitive to the protective effect of Visomitin. The data presented in Table 1 indicate that the
taining eye drops Visomitin (experimental group) or sim ilar drops but without SkQ1 (control group) were admin istered for two weeks. After 15 days, we compared the state of the retina in these groups to determine whether Visomitin has a therapeutic effect on the retina after the illumination of animals. Table 2 summarizes the results of quantitative evaluation of the late pathological changes in retinal samples from the control and experimental ani mals demonstrating the pronounced protective effect of Visomitin on the state of both outer and inner retina after lightinduced damage. Measuring the areas of ONL atrophy in the experi mental and control samples with the most pronounced changes (two samples from each group) showed that administration of Visomitin results in the significant reduction in the areas of ONL atrophy. Evaluation of the atrophic area size in this layer in experimental samples 15 days after illumination shows virtually complete absence of atrophic phenomena (Fig. 4). Visomitin also protects the inner segments of photoreceptor cells of the dorso central retinal area, which serve as targets for light induced damage from photoinduced shortening: the length of these segments in the experimental samples was 10 ± 1 μm, and in the control samples — 7 ± 1 μm. Visomitin caused threefold greater preservation of RPE compared to the control samples (Table 2). According to these criteria, Visomitin has a pronounced therapeutic effect on the outer retina of rats, and its administration largely prevents cell death and local RPE destruction. The inner retina of placeboreceiving rats demon strated pronounced changes in cytoarchitectonics, including the formation of channels to drain the edema same holds true for the late changes in RPE and inner retina. Areas of hypertrophy of the processes of Müller glial cells associated with the areas of ONL damage were present in the INL of the control samples (Fig. 3), where as in the experimental samples reactive Müller gliosis was far less pronounced. This is also true for the cases of such severe changes as the development of channels and microneuromas observed in the majority of control, but not in experimental retinal samples (Table 1). Therapeutic effect of Visomitin SkQ1containing eye drops on retina of albino rats exposed to illumination with bright light. In this series of the experiments, the rats were first illuminated with bright light, and then SkQ1con fluid and microneuromas, six times more often than the retina of experimental samples (Table 2). Thus, Visomitininduced improvement of the state of the outer retina led to the reduction of pathological processes in the areas of the inner retina. It should be added that solitary lymphocytic inflam matory infiltrates were found in the choroid of control, but not in the experimental samples of the eye posterior segment (not shown). DISCUSSION The LIRD (lightinduced retinal degeneration) model is often used when studying lightdependent dam ages of the retinas of animals [17, 18]. According to this model, albino rat eyes are exposed to shortterm illumina tion with light of high intensity (20003000 lx) or long term illumination with light of low or medium intensity (200500 lx) [20, 21]. Albino rats are used for the experi ments because the light sensitivity of their retina is greater than that of pigmented animals due to the absence of melanin pigment in the albino eyes (melanin protects reti nal structures against excessive light) [20, 22]. The uniqueness of the LIRD model is that in addition to the early damages in photoreceptor cells [24], it presents delayed damages in the RPE–Bruch’s membrane– choroid complex and even later compensatoryadaptive processes in the inner retinal layers [23]. Since intense illumination causes rather fast changes in retinal morphol ogy, histological study of eye sections is commonly per formed when using the LIRD model [17, 21, 23]. The degree of retinal damage in the different versions of the LIRD model varies and depends on a number of factors such as light intensity, exposure time, light source, as well as line, gender, age, and body temperature of the animals [17, 29]. For example, it is better to use female rats as their retinas are more sensitive to lightinduced damage [29]. The dynamics of the destructive changes in the two major retinal cell populations serving as targets for light effects (photoreceptors and RPE cells) can also vary [30]. Numerous studies have been dedicated to research on molecular and cellular mechanisms that underlie the pho toinduced damage of retinal cells [31] and the search for compounds that could prevent or at least reduce such damages. Since oxidative stress is an important compo nent of the pathogenesis of photoinduced retinal damage, this search is mainly focused on compounds with antioxi dant properties. For example, it was shown that curcumin and the green tea component epigallocatechin gallate, when used perorally, could reduce the risk of rat retina damage caused by bright light [32, 33]. Synthetic antioxi dants such as TEMPOLH can also protect photoreceptor cells, reducing the level of lipid peroxidation in them [34]. It has been demonstrated on a large number of in vitro and in vivo models that penetrating cation SkQ1 has high antioxidant activity [1214]. However, the mecha nism of SkQ1 effect on eye retina damaged by bright light remained unknown. We investigated this question in two series of experiments with the LIRD model [17, 18] using Visomitin SkQ1containing eye drops. In the first series, when we studied the preventive effect of Visomitin on the retina, albino rats received Visomitin for two weeks, then they were illuminated with bright light and the morphol ogy of their retina was analyzed 3 h and 15 days after the illumination. In the second series, when we studied the therapeutic effect of Visomitin on the retina, rats were first illuminated with bright light, then they received Visomitin for two weeks, and then the morphology of their retinas was analyzed. In both cases, the control ani mals received eye drops of the same composition but without SkQ1 following the same protocols. Experiments on the preventive effect of Visomitin have shown (Table 1 and Figs. 2 and 4) that these eye drops have a pronounced protective effect on the albino rat retina, reducing the risk of the formation of early lightinduced pathological changes and preventing their further development. Visomitin also inhibits the expres sion of late lightinduced retinal changes, in particular, reactive gliosis, which increases the number and thickness of the processes of Müller glia cells. These results are con sistent with data found in literature according to which proliferative activity of Müller glia cells and their specific density is increased in INL areas adjacent to outer retinal damages [35, 36]. Glia is known to be involved in the pro duction and transport of a number of metabolites and neurotrophic factors in the retina [37]. It is also possible that retinal glial cells can be transformed into poorly dif ferentiated precursors capable of differentiating into reti nal neurons under certain conditions [38]. It can be assumed that retinal gliosis described in our experiments can serve as a compensatory event, and Visomitincaused reduction in gliosis level reflects the lower level of photo damage. According to the literature, lightinduced damage of the retina (which is normally an immuneprivileged zone) disturbs the blood–retinal barrier [39], resulting in the possibility of inflammatory infiltration of the retina [40]. In our experiments, illumination of the control rats led to the development of solitary lymphocytic infiltrates in the choroid of their retinas, which indicated damage to the blood–retinal barrier. Visomitin administration pre vented the formation of such infiltrates, indicating the protective effect of the eye drops on this barrier. Experiments studying the therapeutic effect of Visomitin have shown (Table 2 and Fig. 4) that these eye drops reduce the risk of the development of late light induced pathological changes in the retina and lead to the preservation of the population of photoreceptor cells. Photoinduced death of these cells is known to occur not at the moment of illumination, but gradually over some time. During subsequent dark adaptation, photoreceptor cells start entering caspasedependent apoptosis [41], leading to compensatory pathological changes in RPE [18]. The described therapeutic effect of Visomitin on the lightdamaged retina can be explained by the protection of photoreceptor cells against apoptosis. In particular, the data obtained on cell cultures support this hypothesis. According to these data, SkQ and other mitochondria addressed antioxidants prevent photoinduced apoptosis of HeLa cells by reducing the level of oxidative stress in them [42, 43]. It is also possible that the therapeutic effect of SkQ1 is connected with the restoration of the outer segments of some photoreceptor cells after their damage. For example, there is some evidence of the possibility of DNA repair in photoreceptor cells and reversibility of lightinduced changes [44]. Moreover, restoration of outer photoreceptor segments in rats [4547] and humans [48] is possible even in case of complete destruction of these photoreceptor segments as long as their perikaryon and cell nuclei remain intact. These observations suggest that Visomitin SkQ1containing eye drops will be an effective medication to stimulate restoration mechanisms of retinal cells. The described degenerative and atrophic light induced retinal damages are typical for LIRD models in their nature and rate of development [17, 18, 2024]. These damages were of multifocal character, being pres ent in both peripheral and central retinal areas. The dorsocentral retinal area was shown to be particularly prone to lightinduced damage, which is consistent with its maximal sensitivity to light in rats and with data on topographic studies on the LIRD model [29, 49]. The dorsocentral area of the rat retina can be considered as a functional analog of the human retinal macula, the area of sharpest vision. In this context, it is particularly impor tant to note that etiology and mechanisms of animal reti na damage under the conditions of the LIRD model have much in common with the mechanisms of the develop ment of agerelated retinal diseases. For this reason, the LIRD model is widely used in research on new approach es to prevention and treatment of agerelated eye diseases [50], in particular, agerelated macular degeneration, AMD [24, 33, 51], since constant exposure of the human retina to sunlight is considered to be one of the factors contributing to the development of AMD [4, 52, 53]. The data of the presented study indicate the ability of Visomitin SkQ1containing eye drops to have pro nounced preventive and therapeutic effects on the retina of rats exposed to bright light. Moreover, taking into account the previously demonstrated therapeutic effect of SkQ1 on AMD development in rapidly aging OXYS rats [54, 55], Visomitin can be considered as a potential med ical preparation for the prevention and treatment of human retina damages, in particular, those accompany ing agerelated retinal macular degeneration. The authors express their gratitude to Academician V. P. Skulachev for fruitful discussion of the results of this study. This work was financially supported by the Research Institute of Mitoengineering, Lomonosov Moscow State University.
SkQ1: The Road from Laboratory Bench to the Market
url: https://link.springer.com/chapter/10.1007/978-3-319-73344-9_28
Abstract
SkQ1 and other mitochondria-targeted plastoquinone derivatives are promising drug candidates for the treatment of pathologies associated with mitochondrial dysfunction. Experiments in animals revealed that SkQ alleviates damage induced by ischemia-reperfusion injury, prevents autoimmune arthritis, suppresses the development of Alzheimer’s disease signs, inhibits the development of ophthalmological disorders, including dry eye syndrome (DES), and has anti-inflammatory activity. The first SkQ-based drug (Visomitin eye drops) is already developed and registered in Russia; clinical studies of orally administered drug is in progress. In this review, we summarize recent experimental data on SkQ effects in animals, results of clinical trials and future perspectives of mitochondria-targeted antioxidants as therapeutic compounds.
Keywords SkQ1 Mitochondria-targeted plastoquinone derivatives Drug development Dry eye syndrome Visomitin Clinical trials
Introduction The history of mitochondria-targeted compounds dates back to late 1960s, when Skulachev and Liberman performed first experiments with membranophilic penetrating cations (MPCs) on mitochondria and submitochondrial particles (Liberman et al. 1969; Bakeeva et al. 1970; Feniouk and Skulachev 2018). At the
end of 1990s the first MPC-conjugated antioxidants that were able to specifically accumulate in over 1000-fold excess in mitochondria (especially in those with high a Δψ) and to mildly de-energize the mitochondrial membrane were developed. The obvious advantage of such antioxidants consists in extremely low effective concentrations due to their ability to selectively accumulate in the inner mitochondrial membrane. For potential drug candidates, this property allows to greatly diminish the dose applied and the risks of unwanted side effects. At the beginning of this millennium another important step on the way of mitochondria-targeted antioxidants design was made: MitoQ, an MPC conjugated with ubiquinone, was developed by Murphy et al. (Kelso et al. 2001). The important advantage of MitoQ was its ability to be regenerated from the oxidized form back to the reduced one by accepting electrons from the respiratory chain (James et al. 2005), i.e. it was a rechargeable mitochondrial antioxidant. This means that each MitoQ molecule can neutralize numerous ROS species, and therefore such drugs can be applied in even lower doses. It also allows to use the more stable oxidized form of the drug, because it will eventually be reduced in mitochondria, yielding the active anti-oxidant quinole form. Later studies performed in the group of Skulachev on model systems, mitochondria, and cell cultures indicated that ubiquinone, although being a natural mitochondrial electron carrier, is not the best option for the antioxidant moiety for mitochondria-targeted ROS quenchers. MPCs conjugated with plastoquinone, an electron carrier from the chloroplasts, proved to be more promising antioxidants than MitoQ (Feniouk and Skulachev 2018). It must be noted that all quinone-based antioxidants exhibit pro-oxidant activity at high concentrations. However, the anti- and pro-oxidant concentrations of MitoQ are rather close to each other (~300 and ~500 nM, respectively), while for the plastoquinone conjugates this difference was almost 32-fold (~25 and ~800 nM) (Antonenko et al. 2008; Bakeeva et al. 2008; Skulachev et al. 2009). These observations were the starting point for the SkQ-project — a multidisciplinary study of MPCs (or “Sk” — Skulachev ions, as they were named in 1974 by Green (1974)) conjugated to plastoquinone (abbreviated to “Q”) as potential drugs for treatment of pathologies related to oxidative stress, mitochondrial dysfunction, and aging. At the initial stage of the project, we analyzed a broad spectrum of SkQ compounds properties and activities in artificial lipid membranes, in isolated mitochondria, in cell cultures (Antonenko et al. 2008), and in animal models (summarized in Skulachev et al. 2011; Pasyukova et al. 2017; Feniouk and Skulachev 2018). The effects of SkQ compounds on age-related retinal pathologies proved to be the most striking results obtained in the pre-clinical studies. Oxidative stress is an important damaging factor in the retina, because it contains a lot of polyunsaturated fatty acids and because the oxygen concentration in that site is near-arterial, i.e., much higher than in the great majority of other tissues (Beatty et al. 2000; Kanda et al. 2007). Numerous indications of a crucial role of ROS in age-related ocular pathologies, as well as the results we obtained in OXYS rats (an animal model suffering from increased sensitivity to oxidative stress and developing cataract and retinopathies as early as 2.5–3 months of age (Sergeeva et al. 2006; Kolosova et al. 2006)) suggested that eye pathologies are probably a promising line for SkQ application. B. A. Feniouk and M. V. Skulachev 639 Experiments showed (Neroev et al. 2008; Skulache Experiments showed (Neroev et al. 2008; Skulachev et al. 2009) that 250 nmol SkQ1/kg per day consumed with food completely prevented the development of retinopathy and cataract in OXYS rats up to the age of 2 years (Skulachev et al. 2011; Saprunova et al. 2012). Later it was found that such effect can be achieved with local administration of SkQ1 in the form of eye-drops (Neroev et al. 2008). Further development of SkQ1 as potential pharmaceutical split into two major directions: ophthalmic (development of eye-drops) and systemic (development of oral and injectable forms). 2 SkQ1 in Ophthalmics A stable eye-drop form of SkQ1 called «Visomitin» was developed in 2009. Preclinical studies confirmed safety and efficacy of Visomitin eye drops in several animal models of age-related eye diseases, such as cataract, retinopathy (Neroev et al. 2008), glaucoma (Iomdina et al. 2015) and dry eye syndrome (ORA inc, unpublished). Experiments performed on rats in the group of Prof. Kolosova revealed several aspects of SkQ1 mechanism of action in eye pathologies: it slowed down the lacrimal gland degeneration (Bakeeva et al. 2016), normalized the lens metabolome, and the chaperone crystalline activity (Yanshole et al. 2015) and expression (Muraleva et al. 2014; Rumyantseva et al. 2015), ameliorated the pathological gene expression alterations in retina in animals suffering from AMDlike retinopathy (Perepechaeva et al. 2014). Electron microscopy studies demonstrated that SkQ1 given daily with food at the dose of 250 nmol/kg for 5 months, starting from the age of 19 months, prevented such age-dependent destructive processes in retina and vascular layer of eyes as accumulations of lipofuscin granules, and flattening, overgrowing, and degradation of endothelial cells of choriocapillaries (Saprunova et al. 2012). Oral SkQ1 administration to OXYS rats led to an increase in the concentration of mRNA and in the expression of vascular endothelial growth factor A (VEGF, a key regulator of angiogenesis) in retina up to the levels corresponding to the Wistar rats (Markovets et al. 2011). Further clinical studies with dry eye syndrome (DES) carried out on 240 patients for 45 days confirmed the beneficial effect of SkQ1 in humans (Brzheskiy et al. 2015). This double-blinded placebo-controlled study also included a 45 days post-treatment period. The study revealed that SkQ1 reduced such DES symptoms as eye dryness, burning, grittiness, and blurred vision. Importantly, SkQ1 promoted faster corneal wound healing, especially in the subgroup of patients with heavy initial DES (Fig. 1). Similar results were obtained in US Phase II clinical trial that utilized novel system of anti-DES drugs investigation, Controlled Adverse Environment (CAE) challenge (Ousler et al. 2005). In this study, two variants of SkQ1-based eye-drops were tested versus placebo («artificial tear», same as in a previous clinical study) on 90 patients (30 subjects per arm). The first variant was the same «Visomitin» preparation containing 250 nM SkQ1 as in a previous DES study, the second contained tenfold more (2500 nM) SkQ1. The experiment showed higher tolerability (followed with corneal damage and DES symptoms) of CAE challenge in group of Visomitin comparing to placebo (Petrov et al. 2016). formed on 80 patients with senile cataract. In this study, «Visomitin» administration period was 6 months. The trial confirmed long-term safety of ocular SkQ1 administration in humans. The study revealed statistically significant effect of SkQ1 on non-corrected visual acuity of patients in «Visomitin» group compared with placebo group. Importantly, measurements of tear antioxidant activity showed a strong, statistically significant increase in «Visomitin» group only (Erichev et al. 2016). «Visomitin» is now approved and marketed as an Rx drug in Russia. Already over one million doses were sold here in the drug stores. FDA approval process is ongoing in the US.
Systemic Use of SkQ1 In parallel with the development of «Visomitin» in ophthalmics, several preclinical studies were performed with SkQ1 (and its close analog SkQR1) administered systemically. The results of these studies are summarized in Table 1. Conclusions SkQ1 and other mitochondria-targeted plastoquinones are a promising and efficient means to alleviate several age-related pathologies. In case of dry eye syndrome and cataract it is already successfully implemented as a drug (eye drops); clinical trials of the form for oral administration are currently running.
Evaluating the Stability of a Cationic Plastoquinone Derivative (PDTP) in Visomitin Eye Drops
url: https://link.springer.com/article/10.1007%2Fs11094-013-0932-3
Plastoquinonyldecyltriphenylphosphonium bromide (PDTP) belongs to a new generation of targeted antioxidants and contains a hydrophobic cation, the structure of which is shown in Fig. 1. PDTP in clinical trials showed high biological activity [1, 2]. Visomitin eye drops, which have passed clinical trials and are recommended for treating dry eye syndrome, were developed based on PDTP [3]. Preclinical trials showed the effectiveness of the developed eye drops for treating other age-related eye diseases [4–8]. Unfortunately, the shelf life of the developed eye drops turned out to be limited, i.e., one year at storage temperature 4–8°C. This was related to the instability of the active ingredient PDTP. The stability of drugs is known to be affected by many factors, namely, temperature, light, moisture, atmospheric oxygen, and solution pH [9]. For eye drops, only the first two factors (temperature and light) contribute substantially to the degradation rate of the active ingredient. The action of atmospheric oxygen can be decreased or excluded by using special technologies during the preparation process. The pH value of eye drops is a controlled parameter. It was found in a previous study that the PDTP degradation rate was the same in the pH range 6.3–7.3. Herein we present results from a study of the effects of temperature and illuminance on the degree of degradation and; therefore, on the shelf life of Visomitin eye drops consisting of PDTP (from 0.124 to 0.186 g/mL), benzalkonium chloride (from 0.080 to 0.120 mg/mL), and other excipients (hypromellose, NaCl, sodium phosphate buffer at pH 6.3–7.3). EXPERIMENTAL PART The studied compound PDTP (drug substance) and Visomitin eye drops based on it (Batch 201106–8; cardboard box packaging with black inner side; “white polymer” vials) were supplied by MITOTEH Ltd. Doubly distilled H2 O (Milli-Q, Millipore Advantage A10, France) and CH3 CN (HPLC-gradient grade, Panreac, Spain) were used for HPLC and LC/MS/MS analysis. Other reagents were purchased from Aldrich, Sigma, Acros, and Lancaster (purity class at least chemically pure). Quantitative determination of PDTP in eye drops The HPLC/MS method described previously [10] and FSP “Visomitin, eye drops” were used for quantitative determination of the active ingredient in the eye drops. A solution of internal standard [100 L, PDTP-d15 solution (1 M) in EtOH (96%)] was placed into a dark-glass 10-mL flask, treated with EtOH (50%, 5 mL), stirred for 11 min, treated with eye drops (500 L) and 3-chloroperbenzoic acid solution (100 L, 0.5 M) in EtOH, adjusted to the mark with EtOH (50%), and stirred in an OS-10 orbital shaker (BioSan, Latvia) at 350 rpm for 30 min. Chromatography was performed on a UPLC-MS/MS system consisting of an Acquity chromatograph (Waters, USA) and TQD tandem quadrupole MS-detector (Waters, USA). A diluted solution of the sample (11.2 L, full loop) was analyzed on an Acquity BEH C18 column (0.21 5.0 cm, 1.7 m) at 35°C and flow rate 0.5 mL/min using eluents A (20 mM formic acid in H2 O) and B (20 mM formic acid in CH3 CN) according to the gradient program 40–80% B (4 min), 80–40% B (0.1 min), 40–40% B (0.9 min). The main parameters of the MS-detector were positive-ion electrospray (ES+); multiple reaction monitoring (MRM); source temperature 120°C, vaporizer temperature 450°C; cone potential 55 V; capillary potential 3.0 kV; collision gas (Ar) flow rate 0.18 mL/min; collision energy 50 eV; scan transitions 537.30 > 262.13 and 537.30 > 289.12 for PDTP, 552.40 > 277.20 and 552.40 > 304.30 for PDTP-d15. Data were collected and processed using the MassLynks program (Waters, USA). The PDTP concentration was calculated from the ratio of the peak areas for the drug substance and the internal standard (PDTP-d15) taking into account the dilution factor. Determination of chromatographic purity of PDTP solution by HPLC The chromatographic purity of PDTP solutions was determined at concentrations from 0.5 to 0.01 mg/mL according to FSP “Plastoquinonyldecyltriphenylphosphonium bromide, drug substance” in the section “Related impurities.” Chromatographic analysis was performed using an Agilent 1100 HPLC system (Agilent, USA). Solution (1 L) with a concentration of ~0.5 mg/mL [or a proportionally larger volume for solution of lower concentration, solutions of higher concentration were diluted with EtOH (96%) to a concentration of 0.5 mg/mL] was analyzed over a column [0.46 25 cm, Luna C18(2), 5 m, Phenomenex, USA] at 25°C and flow rate 1.0 mL/min with UV detection at 226 nm. The mobile phase was CH3 CN:H2 O (53:47) containing phosphoric acid (20 mM). Data were collected and processed using the ChemStation program (Agilent, USA). Degree of PDTP degradation by the action of visible light as a function of concentration in EtOH solutions Solutions of PDTP with concentrations 50–0.5 mg/mL in EtOH (96%) and of concentrations 0.1–0.01 mg/mL in EtOH (50%) were prepared because of the low solubility of PDTP in aqueous salt solutions (less than 0.1 mg/mL). Identical volumes of test samples were placed in light-protective plastic tubes and irradiated without caps for 3 h at illuminance 20,000 lux (standard general use illuminator based on Premium LEDs, 12 V, 18 W, 1800 Lm, Tekhnologii Budushchego, Russia). This was equivalent to scattered sunlight. The illuminance intensity was measured with a Testo 540 lux meter (Testo AG, Germany). Then, samples with concentrations 50–1 mg/mL were diluted to concentration 0.5 mg/mL. Samples with concentrations 0.5–0.01 mg/mL and a control sample (0.5 mg/mL) were analyzed without diluting. Prepared samples of 50–0.5 mg/mL were analyzed with injector volume 1 L; of 0.1 mg/mL, 5 L; of 0.05 mg/mL, 10 L; of 0.01 mg/mL, 50 L. Degree of PDTP degradation as a function of wavelength of irradiating light in the range 220–650 nm [PDTP solution (0.4 mM) in aqueous glycerin (20%)] A PDTP solution (0.4 mM) in glycerin:H2 O (1:4, m/m) was prepared in order to achieve sufficient solubility and a composition as close as possible to the aqueous solution. An aliquot of the solution (0.5 mL) was placed into a dark-glass chromatographic vial and illuminated without a cap for 5 min by a directed light beam with the selected wavelength [PTI’s QuantaMasterTM 80 with 75 W xenon lamp (185 to 680 nm), Photon Technology International, Inc., USA]. Degradation kinetics of PDTP in eye drops by visible light Samples in droppers without cardboard packaging were illuminated for 5, 10, and 30 min and 1, 2, and 3 h at illuminance 20,000 lux (standard general use illuminator based on Premium LEDs, 12 V, 18 W, 1800 Lm, Tekhnologii Budushchego, Russia). This was equivalent to scattered sunlight. The illuminance intensity was measured with a Testo 540 lux meter (Testo AG, Germany). Degradation of PDTP in Visomitin eye drops at 4 and 25°C for 1 year in standard secondary packaging Vials of eye drops in standard packaging were stored at room temperature (25°C) and in a refrigerator (Electrolux, Sweden) at 4°C. Three samples each were taken after 1, 3, 6, and 12 months and placed into an MDF-192 freezer (Sanyo, Japan) at –80°C. All samples were thawed after storage for a year. The contents were thoroughly stirred. The PDTP content was determined according to the FSP requirements. RESULTS AND DISCUSSION The degree of PDTP degradation by visible light as a function of concentration was studied in EtOH solutions. Figure 2 shows chromatograms of PDTP solutions in EtOH with various initial concentrations before (control) and after exposure for 3 h at illuminance 20,000 lux. Figure 3 shows a plot of the contents of PDTP and degradation products as functions of solution concentration after exposure for 3 h at illuminance 20,000 lux. It was found that the degree of degradation of EtOH solutions of PDTP through the action of visible light decreased with increasing concentration. Many degradation products were formed without any of them dominating regardless of the solution EtOH concentration. The photolability of PDTP at low concentrations was such that exposure to 60,000 lux h was sufficient to study the degradation kinetics of the eye drops (instead of the recommended 1,200,000 lux h [9]). Degree of PDTP degradation as a function of irradiating light wavelength in the range 220–650 nm [PDTP solution (0.4 mM) in aqueous glycerin (20%)] Figure 4 shows chromatograms of PDTP solution in glycerin (20%) after irradiation by light in the wavelength range 220–650 for 5 min. Figure 5 shows a plot of the chromatographic purity and PDTP solution concentration as functions of irradiating light wavelength. It was found that the accumulation of degradation products correlated satisfactorily with reduced PDTP concentration. The greatest extent of degradation was observed for the wavelength corresponding to the absorption maximum at 340 nm and continued up to 450 nm. Degradation kinetics of PDTP in eye drops by visible light Figures 6 and 7 show plots of the PDTP concentration as a function of light irradiation time of Visomitin drops in dropping vials (illuminance 20,000 lux) in normal and semi-logarithmic coordinates. Figure 8 shows typical chromatograms of Visomitin drops in dropping vials for various durations of irradiation by visible light. Table 1 presents the calculated exposure time leading to degradation of 1% PDTP as a function of illuminance. The illuminance intensity was selected according to the recommended standards [11]. It was found based on the results that the dropping vial material (milk-white polyethylene) transmitted wavelengths of light (340–450 nm) that caused PDTP degradation in the eye drops. Exposure for 46 h to room light (500 lux) and for 23 min to direct sunlight (60,000 lux) led to PDTP degradation of 23.5%. This indicated that additional light-protective packaging was necessary. Because the shelf life of an open vial is one month, the vial can be removed from the lightprotective secondary packaging a maximum of 90 times (30 d, 3 times per day). The total losses would be acceptable if the vial were opened (illuminance 3 yr (38 months) and could be applied to storage conditions in a refrigerator at 2–8°C. Possible losses during transport and storage of eye drops at variable temperatures (at room temperature) were about 1% during one month. Thus, it was found that the shelf life during storage of drops in a refrigerator in light-protective packaging at 2–8°C could be increased to 3 yr. Temperature variations were allowed for up to one month during the whole shelf life without substantial quality degradation (losses of PDTP 1%)
] The first experience of using the drug “Visomitin” in the treatment of “dry eye» url: https://cyberleninka.ru/article/n/pervyy-opyt-ispolzovaniya-preparata-vizomitin-v-terapii-suhogo-glaza
Advances in Development of Rechargeable Mitochondrial Antioxidants
url:https://www.sciencedirect.com/science/article/pii/B9780123946256000106
Alexander N. Lukashev*,† , Maxim V. Skulachev*,{ , Victoria Ostapenko*, Alla Yu. Savchenko} , V.V. Pavshintsev*,{ , Vladimir P. Skulachev
Contents 1. Introduction 252 2. Ischemia–Reperfusion 253 3. Age-Dependent Disorders 254 4. Liver Protection 255 5. Inflammation 256 6. Neurodegenerative Diseases 258 7. Ophthalmic Diseases 260 8. Novel Mitochondrial Antioxidants 262 9. Mild Uncoupling 262 References
Abstract It has been about 15 years since the introduction of the rechargeable mitochondriatargeted antioxidants (RMA). Two major groups have been developing RMA of the MitoQ and SkQ types independently, and many additional trials have been done by other researchers. This has provided solid preclinical evidence of RMA efficacy in various models. Human trials of systemic MitoQ were not followed by further advances, but the safety of MitoQ and, most likely, other RMA in humans has been demonstrated. A prooxidant effect at higher concentrations of RMA was described. For RMA of the SkQ type, a large window between anti- and prooxidant concentrations was observed, which makes SkQs promising as potential medicines. Significant RMA-induced improvements in many diseases that do not have an accepted treatment have been described. This justifies further clinical trials of RMA.
Mitochondria are not just power plants of the cell, but they are among the key players in a number of regulatory pathways activated by stress, acting both as signal commuters and as early victims of adverse effects. Mitochondria are central for the metabolism of reactive oxygen species (ROS), which are crucial for many functions of a cell and of organisms [reviewed in Ref. 1]. It is therefore not surprising that mitochondria were seen as an attractive therapeutic target in numerous diseases and pathological syndromes. In fact, the first mitochondrial drug, the uncoupler dinitrophenol, was introduced over 100 years ago. Dinitrophenol was suggested to reduce body weight. Unfortunately, the drug had to be withdrawn because of some adverse effects.2 It is now obvious that it lacked specificity to mitochondria and affected the potential on all membranes in the cell. It was not until many decades later that basic studies of mitochondria precipitated in concepts of mitochondria-targeted molecules. The two ways to aim for mitochondria are specific targeting peptides3,4 and membranophilic cations.5,6 The first approach is useful to direct proteins, although tetrapeptides containing dimethyltyrosine can also be used as mitochondria-targeted antioxidants7 ; the second is suitable for small molecules. An obvious choice of such small molecules was antioxidants, because they lowered the ROS level. A large fraction of ROS in cells are produced in mitochondria, and mitochondria are also very vulnerable to ROS because they contain a high concentration of cardiolipin, one of the prominent ROS targets, and because they can produce additional ROS upon damage by ROS in a positive-feedback loop.8 To fully benefit from targeting by membranophilic cations, such antioxidants should ideally be rechargeable, i.e., they should not just trap ROS, but become reduced for the next use. These requirements are perfectly fulfilled by quinones that are reduced (and thus recharged after trapping a free radical) in center i of the respiratory chain complex III, localized in the inner leaflet of the inner mitochondrial membrane. The first drug using this concept was MitoQ,9 a fusion of ubiquinone and the membranophilic cation decyltriphenylphosphonium (Fig. 10.1). Later, a similar molecule using plastoquinone instead of ubiquinone was suggested.10 This molecule, termed SkQ1 (Fig. 10.1), was shown to have a much broader therapeutic window. All antioxidants exhibit prooxidative properties at increased concentrations, the difference between antioxidant and prooxidant concentrations being much higher for SkQ1 than for MitoQ.10 These two molecules, SkQ1 and MitoQ, further termed rechargeable mitochondrial-targeted antioxidants (RMA), were introduced over a decade ago and to date have been tested in a number of preclinical and clinical trials, which provided good reliability of the findings. This review summarizes recent advances in these trials and the prospect for the next generation of mitochondria-targeted small molecules.
ISCHEMIA–REPERFUSION The most obvious therapeutic target for antioxidants is the ischemia– reperfusion syndrome, because the respiration burst after recovery of circulation may be even more damaging than ischemia per se. Indeed, mitochondrial antioxidants have been tested in animal models of ischemia– reperfusion. Pretreatment of rats with MitoQ (administered as 500 μM in drinking water, which roughly corresponds to 100 μmol/kg/day) significantly improved recovery of isolated rat heart after ischemia and reperfusion.11 SkQ1 was tested in several models of ischemia and reperfusion.12,13 Rats were pretreated with either SkQ1 or its rhodamine analog SkQR1 (Fig. 10.1). The latter compound has properties of a recyclable mitochondrial antioxidant, similar to SkQ1.10 It is poorly suited for drug development because of limited stability, but it is convenient for experimental work because the rhodamine part allows tracking of the compound by fluorescent microscopy. Pretreatment of rats prior to ischemia–reperfusion of the heart led to significant reduction of postinfarction damage and arrhythmia. In a model of kidney ischemia (one kidney was completely removed, the other one was subjected to 1 h ischemia followed by reperfusion), SkQ1 could completely prevent death of animals (Fig. 10.2A) and significantly reduced biochemical markers of kidney insufficiency in the blood serum In a model of compression ischemia of the brain, SkQ1 reduced the volume of infarction more than twofold (Fig. 10.2B). Unfortunately, this success was hard to translate into clinical trials. First, in all in vivostudies pretreatment was required for the optimal effect of RMA. Such options are rarely available in humans. Second, experimental models are easy to standardize by severity of damage and timing of treatment, and pathological material is readily available for examination. In human patients, it is difficult to get precise readouts. It is likely that this line of research would get a second chance as a nonlisted indication after the drugs are approved for any other condition; however, clinical trials in a scheduled surgical operation setting are also feasible.
. AGE-DEPENDENT DISORDERS The grand concept behind the development of SkQ1 was to find and disable the trigger of senescence, which could be viewed as a suicide program rather than a gradual accumulation of occasional damage. It was suggested that such program might be controlled by mitochondria and mitochondrial ROS, which would thus be a valid target for “antiaging” drugs.15 Indeed, SkQ1 prolonged the life spans of a number of species,14,16–19 decreased manifestations of aging, especially in the OXYS rat lineage showing an accelerated senescence due to permanent oxidative stress,20,21 and prevented or improved a plethora of age-related disorders [reviewed in Ref. 18]. Senescence per se is hardly suitable for clinical trials, and therefore the preclinical and clinical development of SkQ1 concentrated on particular diseases, which also include some age-related pathologies (see below).
LIVER PROTECTION One of the most common clinical applications of conventional antioxidants is treatment of liver diseases, most prominently alcoholic and nonalcoholic fatty liver disease and viral hepatitis. MitoQ administered at 5 or 25 mg/kg/day significantly reduced the level of liver steatosis.22 Our group conducted preclinical trials of SkQ1 in a number of animal models, including alcoholic fatty liver disease and carbon tetrachloride (CCl4) and D-galactosamine (D-gal)-induced toxic hepatitis in Wistar rats (Lukashev A.N., unpublished data). There was no significant effect of SkQ1 in models of acute and chronic alcohol damage, which may be explained by lower doses (10–1250 nmol/kg/day) than those used in the MitoQ study. In other models, there was some beneficial effect of SkQ1 on the biochemical markers of liver damage (level of liver enzymes in blood serum); however, the effect was often not statistically significant and was inconsistent. A more significant result was seen upon pathological examination. SkQ1 reduced the area and severity of dystrophy in the CCl4-induced toxic hepatitis model; the most efficient dose was 1250 nmol (670 μg) per kilogram daily administered starting 7 days before induction of hepatitis. The most pronounced effect of SkQ1 was seen in the D-gal model. D-gal sensitizes hepatocytes to trace qualities of lipopolysaccharide (LPS) that enter the blood from the intestine, and in some models D-gal is actually supplemented with LPS to increase toxicity. In our experiments, 3- to 4-month-old Wistar rats were treated with 500 mg/kg D-gal intraperitoneally. SkQ1 was given per os (via gavage) daily starting 7 days before induction of hepatitis. The rats were sacrificed 24 h after induction of hepatitis. SkQ1 (1250 nmol/kg/day) almost entirely prevented the development of dystrophy and significantly reduced pathological signs of inflammation (Fig. 10.3), which was accompanied by decrease in ALT, AST, and LDH serum levels. The high efficacy of SkQ1 in the D-gal model that relies on the damaging effect of LPS is consistent with the report of a protective effect of MitoQ in an LPS/peptidoglycan model of sepsis.23 There are no published data on preclinical trials of MitoQ in liver disease models. In 2008, there was a clinical trial of MitoQ in hepatitis C patients.24 While the drug significantly reduced the level of AST and ALT, it had no effect on viral replication. The advent of novel direct-action antiviral drugs against hepatitis C25 might eliminate the need for accessory liver protective therapy in hepatitis C, so the future of antioxidant treatment in viral hepatitis is unclear. Nevertheless, RMA certainly hold promise as liver protective drugs
. INFLAMMATION ROS have been implicated in many stages of inflammation. While the extracellular ROS produced by NAPDH oxidases are the key mediators and effectors of inflammation, mitochondria and mitochondrial ROS play important roles in inflammation signaling.26 Since there was a significant theoretical and practical background for use of any antioxidants to treat pathological inflammation, mechanistic and preclinical studies of RMA developed in parallel. Even before the onset of inflammation, RMA can affect the immune status. Long-term administration of SkQ1 in mice delayed senescenceassociated myeloid shift in hematopoiesis (from T cells to neutrophils).27 Another study confirmed that SkQ1 affects hematopoiesis in adult mice. Treatment of mice with doses as low as 5 and 50 nmol (2.6 and 26 μg) SkQ1/kg/day for 21 days slightly decreased the percentage of CD8+ (but not CD4+) cells, and significantly decreased the percentage of granulocytes.28 Therefore, it can be speculated that SkQ1 preconditioned the immune system to be “smart, not strong” and avoid over-reactive response. In line with this theoretical evidence, SkQ1 reduced inflammation in experimental bacterial pyelonephritis (which is often excessive for the clearance of a pathogen and may become the main damaging factor), and one proved mechanism was systemic decrease in neutrophil levels.29 This finding might explain the mechanism underlying reduced pathological signs of inflammation (mainly the neutrophil infiltration) observed in many models. A wide range of SkQ1 doses reduced neutrophil infiltration in liver of mice with D-gal hepatitis (see above). Reduction in initial neutrophil infiltration (7 hours) also underlies the effect of topical or systemic SkQ1 administration, which strongly accelerated wound healing in elder rats.30 Besides a decrease in systemic neutrophil levels, RMA may affect activation of endothelium in inflammation and thus decrease infiltration. It has been shown that SkQ1 prevents TNF-α-induced overexpression of ICAM1 in endothelium in vitro and in vivo, which might decrease binding and extravasation of immune cells.31 However, because this effect was also observed upon treatment with C12TPP, it is likely that it is mediated by the uncoupling effect of SkQ1 (see below). The earliest players in inflammation are dendritic cells (DCs). The NLRP3 inflammasome is the key regulatory complex in activation of DCs, and mitochondria play an important role in activation of the inflammasome.32 It has been shown that MitoQ alleviated symptoms of experimental colitis in mice, at least in part by preventing activation of the NLRP3 inflammasome in DCs and release of IL-1b and IL-18.33 The MitoQ effect consisted of both decrease in transcription of interleukin mRNA and in suppression of caspase-1 involved in their activation; therefore, the effect of RMA on DCs may be multimodal and requires further study. The major therapeutic challenges involving pathological inflammation are autoimmune diseases. MitoQ was shown to improve clinical score in experimental autoimmune encephalomyelitis, a common experimental model of multiple sclerosis. Both pretreatment and treatment regimens were efficacious, and the beneficial effect was attributed to direct neuroprotection rather than suppression of inflammation.34 The effect of SkQ1 in a model of collagen-induced arthritis in rats varied depending on the lineage of the rats and the administration regimen from complete prevention of disease in most animals to significant improvement of some pathological signs (Lukashev et al., manuscript in preparation). After inflammation has already developed, RMA may have the ability to alleviate the unwanted consequences on the organismal level. In a model of acute sepsis in mice induced by injection of LPS and peptidoglycan, MitoQ decreased the level of liver enzymes, IL-6, and IL-10 in the blood.35 Mito-E (vitamin E coupled to TPP for mitochondrial targeting) and melatonin demonstrated even more pronounced beneficial effect in that study
NEURODEGENERATIVE DISEASES
ROS and mitochondria are involved in the pathogenesis of a number of neurodegenerative diseases including Parkinson’s disease (PD), Alzheimer’s disease (AD), Huntington’s disease, and amyotrophic lateral sclerosis (ALS) [reviewed in Ref. 36,37]. Therefore, neurodegenerative disorders are attractive targets for RMA. There are many studies indicating that amyloid beta (Aβ) initiates ROS production in vitroand in vivo, and these ROS primarily originate from mitochondria. It was also found that Aβ accumulates in the mitochondrial matrix and can directly damage mitochondria.38 Both MitoQ and SkQ1 protected neurons from Aβ in brain slices ex vivo.39,40 MitoQ reduced neurological deficit in a triple-transgene model of AD,40 while SkQ1 administered to OXYS rats improved age-related neurological symptoms, which were similar to those seen in AD patients.41 Importantly, in both studies, RMA prevented accumulation of Aβ; therefore, at least a part of their effect was due to slowing disease progression, not just compensation of neurological deficit. The body of preclinical data, the absence of effective therapy, and the burden of AD make it one of the most promising applications for RMA. Mitochondrial dysfunction has also been widely implicated in PD. Ubiquinone, the active part of MitoQ, has been tested in PD in a number of clinical trials [reviewed in Ref. 42]. The effects were beneficial, but limited and poorly reproducible. There are no publications on preclinical testing of RMA in PD. Clinical trials of MitoQ in PD were unsuccessful.43 ALS is another neurodegenerative disease with many implications of mitochondrial involvement.36,44 The most common animal model of ALS is the superoxide dismutase (SOD1) transgenic mouse SOD1- G93A.45 These mice develop early neurological manifestations similar to ALS and die before the age of 5–7 months. MitoQ administered in drinking water from 90 days of age (onset of symptoms) at about 100 μmol/kg/day prolonged survival of SOD1-G93A mice by 6 days (medial) and improved some pathological and clinical signs of the disease.46 SkQ1 was also tested in SOD1-G93A mice. Briefly, we used B6SJL-Tg (SOD1-G93A)dl1Gur/J mice (the Pushchino breeding facility) that usually develop progressive neurological deficit and die at 8–9 months of age. At day 120 (before onset of the disease), the animals were weighed and randomized into groups of 20–22 mice with even sex ratio. Males and females were analyzed separately because of the known sexual dimorphism of this model. SkQ1 was administered in drinking water at 250 nmol/kg/day (i.e. 400 less than MitoQ in a similar model). Neurophysiological parameters were assayed in the open field test weekly. In early disease stages, all behavioral and physiological parameters were significantly improved in the treated group compared to the control (Fig. 10.4). For example, at day 215, the treated female mice demonstrated twice higher mean speed and 20% higher grip strength, and 7% higher mean body weight. Unfortunately, after initial improvement, these parameters progressively deteriorated in the treated group, and before death there was no significant difference from the control group. SkQ1 did not have a significant effect on the life span of the mice, which was 270–310 days for females and 255–290 days for males in all groups. Therefore, SkQ1 did not prolong life of SOD1-G93A mice and did not halt the disease progression, but it significantly improved neurological manifestations and “quality of life” at the medium stage of the disease. The moderate effect of both RMA in the ALS model makes it an unlikely choice for the first clinical trials of RMA as neuroprotectors.
OPHTHALMIC DISEASES ROS have been implicated in many ophthalmic diseases, and it was technically very convenient to test RMA in ophthalmic applications because topical administration avoided many uncertainties of systemic administration of the drugs. SkQ1 administered as eye drops (250 nM solution) was very effective in age-related retinopathy in OXYS rats (Fig. 10.5). In veterinary trials, SkQ1 restored lost vision in 61 of 91 dogs, cats, and horses with recent blindness due to retinopathy, and it improved vision in about 50% animals with impaired vision.14,18,21 This result is especially impressive knowing that the only current efficacious treatment of retinopathy is based on inhibition of vascular endothelial growth factor,47 an expensive treatment with potential side effects. Clinical improvement was also recorded in 102 of 105 animals with uveitis and conjunctivitis. This study might be the basis for clinical trials of SkQ1 in humans. SkQ1 prevented the development of age-related cataract or reduced clinical grade of an already present cataract in OXYS rats (Fig. 10.5).21 A stable pharmaceutical composition of SkQ1 in the form of ophthalmic solution (eye drops) was developed. It was found that SkQ1 solutions in water are unstable at concentrations below 10 μM. However, such solutions were successfully stabilized by addition of another membranophilic cation (benzalconium chloride), hydroxypropyl methylcellulose, and a buffer solution, pH 6.5–6.8. Such eye drops containing 250 nM SkQ1 were termed Visomitin. Further studies showed that all components of Visomitin, including SkQ1, remain stable for at least 12 months when stored at 2–8 C. It was found that SkQ1 in this solution is very sensitive to the light, and therefore, it should be stored in darkness or in dark vials.48 In preclinical trials, Visomitin prevented the development of age-related cataract and reduced clinical grade of an already present cataract in OXYS rats (Fig. 10.5).21 In another series of preclinical studies, Visomitin showed good efficacy in two models of dry eye syndrome (M. V. Skulachev et al., paper in preparation). The first human clinical trials of SkQ1 were set up for dry eye syndrome because it is a common and relatively benign condition, which nevertheless allows studying beneficial effects of the drug. Phase I–II trials compared the efficacy of Visomitin eye drops (155 ng SkQ1/ml, 2 mg hydroxypropyl methylcellulose/ml, accessory compounds) to Tears Naturale manufactured by Alcon (2 mg hydroxypropyl methylcellulose/ml, 1 mg dextran 70/ml, accessory compounds). Over 21 days of treatment, Visomitin almost completely reduced hyperemia and edema of the conjunctiva, while the reference drug had only a marginal effect (Fig. 10.6).49 Visomitin completely healed corneal microerosions compared to a threefold reduction in the control group, and it produced a small (0.03 units) but statistically significant improvement in vision compared to none in the control group. Results of Phase III are currently being prepared for publication. Visomitin eye drops are now marketed in Russia (160,000 vials are already sold by drugstores), and a Phase II trial in dry eye syndrome has been initiated in the United States. It is likely that the list of ophthalmic indications of SkQ1 would be quickly expanded because of its efficacy in retinopathy, cataract, NOVEL MITOCHONDRIAL ANTIOXIDANTS Over the last 15 years, mitochondrial antioxidants have evolved from targeted expendable antioxidants, which were already more efficient than general antioxidants,50 to recyclable antioxidants that have nanomolar working concentrations and a significantly wide therapeutic window. It has been noted that the antioxidant properties of TPP-linked quinones improved upon substitution of methoxy groups in ubiquinone to methyl or hydrogen.51 This observation led to the development of an SkQ1 analog based on thymoquinone, an herbal antioxidant that underlies the pharmacological activity of black cumin known since biblical times. This molecule, termed SkQT1, exhibited the penetrating and recyclable antioxidant properties, just as SkQ1, but had the same antioxidant activity as SkQ1 at about 10 lower concentration, while its prooxidant properties manifested at concentrations about twice higher than SkQ1.51 Therefore, SkQT1 has a 600-fold theoretical therapeutic window, 30 better than that of SkQ1.51 MILD UNCOUPLING Rechargeable mitochondrial antioxidants efficiently decrease the production of mitochondrial ROS level. Another strategy to achieve this goal is mild uncoupling, i.e., partial decrease in the electric potential on the mitochondrial membrane.52 A number of membranophilic ions can perform this function, and it turned out that C12-TPP and C12-rhodamine, the transporter parts of RMA molecules, also decrease electric potential in mitochondria even in the absence of the quinone part.53 In line with this theoretical evidence, C12-rhodamine protected kidneys from damage in ischemia–reperfusion and rhabdomyolysis mouse models, and brain in an ischemia–reperfusion model, although it was less potent than SkQR1.54 Therefore, mild uncoupling may add to the mitochondrial protection exhibited by RMA, and it is currently not known which of the reported effects of RMA can be attributed to uncoupling rather than direct antioxidant effect. In future, it may be recommended to include a membranophilic cation backbone (C12TPP or C12-rhodamine) as a control in basic experiments with RMA. As this effect is synergistic with the direct antioxidant action in most settings, this requirement may not be necessary for preclinical studies.
For search engines
Eye treatments. Vision improvements. Eyes, Researches, The science, Scientific breakthroughs in the world, Lenses, Glaucoma, Age-related macular degeneration, Dry eye syndrome (DES), Non-infectious uveitis. Cataract Treatment. Cataract. Best Eye Treatment Google Scholar PubMed Scopus Web of Science ScienceDirect Baidu SciELO EBSCOHost doaj Directory of Open Access Journals Academia Springer Science+Business Media Elsevier Thieme Medical Publishers ResearchGate Open Library Wiley Online Library Palgrave Macmillan Cambridge University Press Oxford University Press Routledge Axel Springer SE Emerald Group Publishing Princeton University Press Yale University Press MIT Press Academic Press Taylor and Francis CRC Press McGraw Hill Education Barnes and Noble Pearson Education BBC British Broadcasting Corporation Harvard University Random House SAGE Publications HarperCollins Imperial College London safe eye treatment
Tag Cloud:
Albania, Algeria, Andorra, Angola, Antigua and Barbuda, Argentina, Armenia, Australia, Austria, Azerbaijan, Bahamas, Bahrain, Bangladesh, Barbados, Belarus, Belgium, Belize, Benin, Bhutan, Bolivia, Bosnia and Herzegovina, Botswana, Brazil, Brunei, Bulgaria, Burkina Faso, Burundi, Côte d’Ivoire, Cabo Verde, Cambodia, Cameroon, Canada, Central African Republic, Chad, Chile, China, Colombia, Comoros, Congo (Congo-Brazzaville), Costa Rica, Croatia, Cuba, Cyprus, Czechia (Czech Republic), Democratic Republic of the Congo, Denmark, Djibouti, Dominica, Dominican Republic, Ecuador, Egypt, El Salvador, Equatorial Guinea, Eritrea, Estonia, Eswatini (fmr. “Swaziland”), Ethiopia, Fiji, Finland, France, Gabon, Gambia, Georgia, Germany, Ghana, Greece, Grenada, Guatemala, Guinea, Guinea-Bissau, Guyana, Haiti, Holy See, Honduras, Hungary, Iceland, India, Indonesia, Iran, Iraq, Ireland, Israel, Italy, Jamaica, Japan, Jordan, Kazakhstan, Kenya, Kiribati, Kuwait, Kyrgyzstan, Laos, Latvia, Lebanon, Lesotho, Liberia, Libya, Liechtenstein, Lithuania, Luxembourg, Madagascar, Malawi, Malaysia, Maldives, Mali, Malta, Marshall Islands, Mauritania, Mauritius, Mexico, Micronesia, Moldova, Monaco, Mongolia, Montenegro, Morocco, Mozambique, Myanmar (formerly Burma), Namibia, Nauru, Nepal, Netherlands, New Zealand, Nicaragua, Niger, Nigeria, North Korea, North Macedonia, Norway, Oman, Pakistan, Palau, Palestine State, Panama, Papua New Guinea, Paraguay, Peru, Philippines, Poland, Portugal, Qatar, Romania, Russia, Rwanda, Saint Kitts and Nevis, Saint Lucia, Saint Vincent and the Grenadines, Samoa, San Marino, Sao Tome and Principe, Saudi Arabia, Senegal, Serbia, Seychelles, Sierra Leone, Singapore, Slovakia, Slovenia, Solomon Islands, Somalia, South Africa, South Korea, South Sudan, Spain, Sri Lanka, Sudan, Suriname, Sweden, Switzerland, Syria, Tajikistan, Tanzania, Thailand, Timor-Leste, Togo, Tonga, Trinidad and Tobago, Tunisia, Turkey, Turkmenistan, Tuvalu, Uganda, Ukraine, United Arab Emirates, United Kingdom, United States of America, Uruguay, Uzbekistan, Vanuatu, Venezuela, Vietnam, Yemen, Zambia, Zimbabwe, USA, North America, South America, Europe
United States / USA
Alabama Alaska Arizona Arkansas California Colorado Connecticut Delaware Florida Georgia Hawaii Idaho Illinois Indiana Iowa Kansas Kentucky Louisiana Maine Maryland Massachusetts Michigan Minnesota Mississippi Missouri Montana Nebraska Nevada New Hampshire New Jersey New Mexico New York North Carolina North Dakota Ohio Oklahoma Oregon Pennsylvania Rhode Island South Carolina South Dakota Tennessee Texas Utah Vermont Virginia Washington West Virginia Wisconsin Wyoming
Alameda Albany Berkeley Dublin Emeryville Fremont Hayward Livermore Newark Oakland Piedmont Pleasanton San Leandro Union City Amador Ione Jackson Plymouth Sutter Creek Biggs Biggs Chico Gridley Oroville Paradise Angels Camp Colusa Williams Antioch Brentwood Clayton Concord Danville El Cerrito Hercules Lafayette Martinez Moraga Oakley Orinda Pinole Pittsburg Pleasant Hill Richmond San Pablo San Ramon Walnut Creek Crescent City Placerville South Lake Tahoe Clovis Coalinga Firebaugh Fowler Fresno Huron Kerman Kingsburg Mendota Orange Cove Parlier Reedley Sanger San Joaquin Selma Orland Willows Arcata Blue Lake Eureka Ferndale Fortuna Rio Dell Trinidad Brawley Calexico Calipatria El Centro Holtville Imperial Westmorland Bishop Arvin Arvin Bakersfield California City Delano Maricopa Mcfarland Ridgecrest Shafter Taft Tehachapi Wasco Avenal Corcoran Hanford Lemoore Clearlake Lakeport Susanville Agoura Hills Alhambra Arcadia Artesia Avalon Azusa Baldwin Park Bell Bellflower Bell Gardens Beverly Hills Bradbury Burbank Calabasas Carson Cerritos Claremont Commerce Compton Covina Cudahy Culver City Diamond Bar Downey Duarte El Monte El Segundo Gardena Glendale Glendora Hawaiian Gardens Hawthorne Hermosa Beach Hidden Hills Huntington Park Industry Inglewood Irwindale La Canada Flintridge La Habra Heights Lakewood La Mirada Lancaster La Puente La Verne Lawndale Lomita Long Beach Los Angeles Lynwood Malibu Manhattan Beach Maywood Monrovia Montebello Monterey Park Norwalk Palmdale Palos Verdes Estates Paramount Pasadena Pico Rivera Pomona Rancho Palos Verdes Redondo Beach Rolling Hills Rolling Hills Estates Rosemead San Dimas San Fernando San Gabriel San Marino Santa Clarita Santa Fe Springs Santa Monica Sierra Madre Signal Hill South El Monte South Gate South Pasadena Temple City Torrance Vernon Walnut West Covina West Hollywood Westlake Village Whittier Chowchilla Madera Belvedere Corte Madera Fairfax Larkspur Mill Valley Novato Ross San Anselmo San Rafael Sausalito Tiburon Fort Bragg Point Arena Ukiah Willits Atwater Dos Palos Gustine Livingston Los Banos Merced Alturas Mammoth Lakes Carmel-By-The-Sea Del Rey Oaks Gonzales Greenfield King City Marina Monterey Pacific Grove Salinas Sand City Seaside Soledad American Canyon Calistoga Napa St Helena Yountville Grass Valley Nevada City Truckee Aliso Viejo Anaheim Brea Buena Park Costa Mesa Cypress Dana Point Fountain Valley Fullerton Garden Grove Huntington Beach Irvine Laguna Beach Laguna Hills Laguna Niguel Laguna Woods La Habra Lake Forest La Palma Los Alamitos Mission Viejo Newport Beach Orange Placentia Rancho Santa Margarita San Clemente San Juan Capistrano Santa Ana Seal Beach Stanton Tustin Villa Park Westminster Yorba Linda Auburn Colfax Lincoln Loomis Rocklin Roseville Portola Banning Beaumont Blythe Calimesa Canyon Lake Cathedral City Coachella Corona Desert Hot Springs Eastvale Hemet Indian Wells Indio Jurupa Valley Lake Elsinore La Quinta Menifee Moreno Valley Murrieta Norco Palm Desert Palm Springs Perris Rancho Mirage Riverside San Jacinto Temecula Wildomar Citrus Heights Elk Grove Folsom Galt Isleton Rancho Cordova Sacramento Hollister San Juan Bautista Adelanto Apple Valley Barstow Big Bear Lake Chino Chino Hills Colton Fontana Grand Terrace Hesperia Highland Loma Linda Montclair Needles Ontario Rancho Cucamonga Redlands Rialto San Bernardino Twentynine Palms Upland Victorville Yucaipa Yucca Valley Carlsbad Chula Vista Coronado Del Mar El Cajon Encinitas Escondido Imperial Beach La Mesa Lemon Grove National City Oceanside Poway San Diego San Marcos Santee Solana Beach Vista San Francisco Escalon Lathrop Lodi Manteca Ripon Stockton Tracy Arroyo Grande Atascadero El Paso De Robles Grover Beach Morro Bay Pismo Beach San Luis Obispo Atherton Belmont Brisbane Burlingame Colma Daly City East Palo Alto Foster City Half Moon Bay Hillsborough Menlo Park Millbrae Pacifica Portola Valley Redwood City San Bruno San Carlos San Mateo South San Francisco Woodside Buellton Carpinteria Goleta Guadalupe Lompoc Santa Barbara Santa Maria Solvang Campbell Cupertino Gilroy Los Altos Los Altos Hills Los Gatos Milpitas Monte Sereno Morgan Hill Mountain View Palo Alto San Jose Santa Clara Saratoga Sunnyvale Capitola Santa Cruz Scotts Valley Watsonville Anderson Redding Shasta Lake Loyalton Dorris Dunsmuir Etna Fort Jones Montague Mount Shasta Tulelake Weed Yreka Benicia Dixon Fairfield Rio Vista Suisun City Vacaville Vallejo Cloverdale Cotati Healdsburg Petaluma Rohnert Park Santa Rosa Sebastopol Sonoma Windsor Ceres Hughson Modesto Newman Oakdale Patterson Riverbank Turlock Waterford Live Oak Yuba City Corning Red Bluff Tehama Dinuba Exeter Farmersville Lindsay Porterville Tulare Visalia Woodlake Sonora Camarillo Fillmore Moorpark Ojai Oxnard Port Hueneme Santa Paula Simi Valley Thousand Oaks Ventura Davis West Sacramento Winters Woodland Marysville Wheatland
Cejkova J, et al. Ocular surface injuries in autoimmune dry eye. The severity of microscopical disturbances goes parallel with the severity of symptoms of dryness. Histol Histopathol. 2009;24(10):1357–65. 2. Seen S, Tong L. Dry eye disease and oxidative stress. Acta Ophthalmol. 2018;96(4):e412–e420. https:// doi.org/10.1111/aos.13526 3. McMonnies C. Reactive oxygen species, oxidative stress, glaucoma and hyperbaric oxygen therapy. J Optom. 2018;11(1):3–9. https://doi.org/10.1016/j. optom.2017.06.002 4. Wakamatsu TH, et al. Evaluation of lipid oxidative stress status in Sjogren syndrome patients. Invest Ophthalmol Vis Sci. 2013;54(1):201–10. 5. Wakamatsu TH, Dogru M, Tsubota K. Tearful relations: oxidative stress, inflammation and eye diseases. Arq Bras Oftalmol. 2008;71(6 Suppl):72–9.
Ward SK, et al. The role of oxidative stress and inflammation in conjunctivochalasis. Invest Ophthalmol Vis Sci. 2010;51(4):1994–2002. 7. Wakamatsu TH, et al. Evaluation of lipid oxidative stress status and inflammation in atopic ocular surface disease. Mol Vis. 2010;16:2465–75. 8. Uchino Y, et al. Oxidative stress induced inflammation initiates functional decline of tear production. PLoS One. 2012;7(10):e45805. 9. Nita M, Grzybowski A. The role of the reactive oxygen species and oxidative stress in the pathomechanism of the age-related ocular diseases and other pathologies of the anterior and posterior eye segments in adults. Oxid Med Cell Longev. 2016;2016:3164734. 10. Stevenson W, Chauhan SK, Dana R. Dry eye disease: an immune-mediated ocular surface disorder. Arch Ophthalmol. 2012;130(1):90–100. 11. Pflugfelder SC, Stern ME, Symposium P. Immunoregulation on the ocular surface: 2nd cullen symposium. Ocul Surf. 2009;7(2):67–77. 12. Wei Y, Asbell PA. The core mechanism of dry eye disease is inflammation. Eye Contact Lens. 2014;40(4):248–56. 13. Baudouin C, et al. Clinical impact of inflammation in dry eye disease: proceedings of the ODISSEY group meeting. Acta Ophthalmol. 2018;96(2):111– 119. https://doi.org/10.1111/aos.13436 14. Craig JP, et al. TFOS DEWS II definition and classification report. Ocul Surf. 2017;15(3):276–83. 15. Wang L, et al. Concentration-dependent effects of transforming growth factor beta1 on corneal wound healing. Mol Vis. 2011;17:2835–46. 16. Liu XF, et al. Nrf2, a potential therapeutic target against oxidative stress in corneal diseases. Oxid Med Cell Longev. 2017;2017:2326178. 17. Deng R, et al. Oxidative stress markers induced by hyperosmolarity in primary human corneal epithelial cells. PLoS One. 2015;10(5):e0126561. 18. Terai K, et al. Crosstalk between TGF-beta and MAPK signaling during corneal wound healing. Invest Ophthalmol Vis Sci. 2011;52(11):8208–15. 19. Chen L, et al. IkappaB kinase beta regulates epithelium migration during corneal wound healing. PLoS One. 2011;6(1):e16132. 20. Demianenko IA, et al. Novel mitochondria-targeted antioxidants, ‘‘Skulachev-ion’’ derivatives, accelerate dermal wound healing in animals. Biochemistry (Mosc). 2010;75(3):274–80. 21. Skulachev VP. Cationic antioxidants as a powerful tool against mitochondrial oxidative stress. Biochem Biophys Res Commun. 2013;441(2):275–9. 22. Zinovkin RA, Bakeeva LE, Chernyak BV, Egorov MV, Isaev NK, Kolosova NG, Korshunova GA, Manskikh VN, Moshkin MP, Plotnikov EY, Rogovin KA, Savchenko AY, Zamyatnin AAJ, Zorov DB, Skulachev MV, Skulachev VP. Mitochondria-targeted antioxidants. Systems biology of free radicals and antioxidants, ed. I. Laher, vol. 2. Berlin, Heidelberg: Springer; 2014. 23. Skulachev VP. Cationic antioxidants as a powerful tool against mitochondrial oxidative stress. Biochem Biophys Res Commun. 2013;441(2):275–9. 24. Nakamura S, et al. Involvement of oxidative stress on corneal epithelial alterations in a blink-suppressed dry eye. Invest Ophthalmol Vis Sci. 2007;48(4):1552–8. 25. Apostolova N, Victor VM. Molecular strategies for targeting antioxidants to mitochondria: therapeutic implications. Antioxid Redox Signal. 2015;22(8):686–729. 26. Dai DF, et al. Mitochondrial oxidative stress in aging and healthspan. Longev Healthspan. 2014;3:6. 27. Demyanenko IA, et al. Mitochondria-targeted antioxidant SkQ1 improves impaired dermal wound healing in old mice. Aging (Albany NY). 2015;7(7):475–85. 28. Chelombitko MA, et al. Comparison of the effects of mitochondria-targeted antioxidant 10-(60 -plastoquinonyl)decyltriphenylphosphonium bromide (SkQ1) and a fragment of its molecule dodecyltriphenylphosphonium on carrageenan-induced acute inflammation in mouse model of subcuteneous air pouch. Bull Exp Biol Med. 2017;162(6):730–3. 29. Demyanenko IA, et al. Mitochondria-targeted antioxidant SkQ1 improves dermal wound healing in genetically diabetic mice. Oxid Med Cell Longev. 2017;2017:6408278. https://doi.org/10.1155/2017/ 6408278 30. Shabalina IG, et al. Improved health-span and lifespan in mtDNA mutator mice treated with the mitochondrially targeted antioxidant SkQ1. Aging. 2017;9(2):315–39. 31. Yang YH, et al. In vivo immunoregulatory properties of the novel mitochondria-targeted antioxidant SkQ1. Mol Immunol. 2012;52(1):19–29.
Petrov A, et al. SkQ1 Ophthalmic solution for dry eye treatment: results of a phase 2 safety and efficacy clinical study in the environment and during challenge in the controlled adverse environment model. Adv Ther. 2016;33(1):96–115. 33. Wei Y, et al. Isoforms of secretory group two phospholipase A (sPLA2) in mouse ocular surface epithelia and lacrimal glands. Invest Ophthalmol Vis Sci. 2012;53(6):2845–55. 34. Hori Y, et al. Effect of retinoic acid on gene expression in human conjunctival epithelium: secretory phospholipase A2 mediates retinoic acid induction of MUC16. Invest Ophthalmol Vis Sci. 2005;46(11):4050–61. 35. Epstein SP, et al. Comparative toxicity of preservatives on immortalized corneal and conjunctival epithelial cells. J Ocul Pharmacol Ther. 2009;25(2):113–9. 36. van Meerloo J, Kaspers GJ, Cloos J. Cell sensitivity assays: the MTT assay. Methods Mol Biol. 2011;731:237–45. 37. Chen D, et al. sPLA2-IIa is an inflammatory mediator when the ocular surface is compromised. Exp Eye Res. 2009;88(5):880–8. 38. Cao L, et al. Downregulation of PTEN at corneal wound sites accelerates wound healing through increased cell migration. Invest Ophthalmol Vis Sci. 2011;52(5):2272–8. 39. Yoon KC, et al. Expression of CXCL9, -10, -11, and CXCR3 in the tear film and ocular surface of patients with dry eye syndrome. Invest Ophthalmol Vis Sci. 2010;51(2):643–50. 40. Saika S, et al. Role of p38 MAP kinase in regulation of cell migration and proliferation in healing corneal epithelium. Invest Ophthalmol Vis Sci. 2004;45(1):100–9. 41. Pruzanski W, et al. Phospholipase A2 in juvenile rheumatoid arthritis: correlation to disease type and activity. J Rheumatol. 1994;21(10):1951–4. 42. Neroev VV, et al. Mitochondria-targeted plastoquinone derivatives as tools to interrupt execution of the aging program. 4. Age-related eye disease. SkQ1 returns vision to blind animals. Biochemistry (Mosc). 2008;73(12):1317–28. 43. Wang Z, et al. Phosphatase-mediated crosstalk control of ERK and p38 MAPK signaling in corneal epithelial cells. Invest Ophthalmol Vis Sci. 2006;47(12):5267–75. 44. Simon HU, Haj-Yehia A, Levi-Schaffer F. Role of reactive oxygen species (ROS) in apoptosis induction. Apoptosis. 2000;5(5):415–8. Dry Eye Workshop (DEWS) Committee. 2007 Report of the International Dry Eye Workshop (DEWS. Ocul Surf. 2007;5(2):65–204. 2. Baudouin C. The pathology of dry eye. Surv Ophthalmol. 2001;45(Suppl 2):S211–20. 3. Yeh S, Song XJ, Farley W, et al. Apoptosis of ocular surface cells in experimentally induced dry eye. Invest Ophthalmol Vis Sci. 2003;44:124–9. 4. Sharma A, Hindman HB. Aging: a predisposition to dry eyes. J Ophthalmol. 2014;2014:781683. 5. Brzhesky BB, Somov EE. Corneal and conjunctival xerosis (diagnosis, clinical features, management). 2nd ed. St. Petersburg: Levsha; 2003. pp 119. 6. Brzheskiy BB, Somov EE. Current treatment of corneal and conjunctival xerosis. Proceedings of the 3rd Russian National School Ophthalmologists. 2004. p. 250–7. 7. Tsubota K, Kawashima M, Inaba T, et al. The antiaging approach for the treatment of dry eye. Cornea. 2012;31(Suppl 1):S3–8. 8. Wakamatsu TH, Dogru M, Tsubota K. Tearful relations: oxidative stress, inflammation and eye diseases. Arq Bras Oftalmol. 2008;71(Suppl 6):72–9. 9. Skulachev VP. Functions of mitochondria: from intracellular power stations to mediators of a senescence program. Cell Mol Life Sci. 2009;66(11–12):1785–93. 10. Korshunov SS, Skulachev VP, Starkov AA. High protonic potential actuates a mechanism of production of reactive oxygen species in mitochondria. FEBS Lett. 1997;416:15–8. 11. Kelso GF, Porteous CM, Coulter CV, et al. Selective targeting of a redox-active ubiquinone to mitochondria within cells: antioxidant and antiapoptotic properties. J Biol Chem. 2001;276:4588–96. 12. Antonenko YN, Avetisyan AV, Bakeeva LE, et al. Mitochondria-targeted plastoquinone derivatives as tools to interrupt execution of the aging program. 1. Cationic plastoquinone derivatives: Synthesis and in vitro studies. Biochemistry (Moscow). 2008;73:1273–87. 13. Skulachev VP, Anisimov VN, Antonenko YN, et al. An attempt to prevent senescence: a mitochondrial approach. Biochim Biophys Acta. 2009;1787:437–61. 14. Skulachev MV, Antonenko YN, Anisimov VN, et al. Mitochondrial-targeted plastoquinone derivatives. Effect on senescence and acute age-related pathologies. Curr Drug Targets. 2011;12:800–26. 15. Neroev VV, Archipova MM, Bakeeva LE, et al. Mitochondria-targeted plastoquinone derivatives as tools to interrupt execution of the aging program. 4. Age-related eye disease. SkQ1 returns vision to blind animals. Biochemistry (Moscow). 2008;73:1317–28. 16. Snytnikova OA, Tsentalovich YP, Stefanova NA, et al. The therapeutic effect of mitochondria-targeted antioxidant SkQ1 and Cistanche deserticola is associated with increased levels of tryptophan and kynurenine in the rat lens. Dokl Biochem Biophys. 2012;447:300–3. 17. Rumyantseva YV, Ryabchikova EI, Fursova AZ, Kolosova NG. Ameliorative effects of SkQ1 eye drops on cataractogenesis in senescence-accelerated OXYS rats. Graefes Arch Clin Exp Ophthalmol. 2015;253(2):237–48. 18. Yani EV, Katargina LA, Chesnokova NB, et al. The first experience of using the drug Vizomitin in the treatment of dry eyes. Pract Med (Russ). 2012;4(59):134–7. 19. Wikipedia. Golovin-Sivtsev table 2015. Available at: https://en.wikipedia.org/wiki/Golovin%E2%80% 93Sivtsev_table. Accessed 21 June 2015. 20. Mo´dis L, Szalai E. Dry eye diagnosis and management. Expert Rev Ophthalmol. 2011;6:67–79. 21. Baudouin C, Aragona P, Van Setten G, et al. Diagnosing the severity of dry eye: a clear and practical algorithm. Br J Ophthalmol. 2014;98(9):1168–76. 22. Zeev MS, Miller DD, Latkany R. Diagnosis of dry eye disease and emerging technologies. Clin Ophthalmol. 2014;8:581–90. 23. Schein OD, Tielsch JM, Munoz B, Bandeen-Roche K, West S. Relation between signs and symptoms of dry eye in the elderly. A population-based perspective. Ophthalmology. 1997;104:1395–401. 24. Foulks GN. Challenges and pitfalls in clinical trials of treatments for dry eye. Ocul Surf. 2003;1:20–30. 25. Nichols KK, Nichols JJ, Mitchell GL. The lack of association between signs and symptoms in patients with dry eye disease. Cornea. 2004;23:762–70. 26. Sullivan BD, Whitmer D, Nichols KK, et al. An objective approach to dry eye disease severity. Invest Ophthalmol Vis Sci. 2010;51:6125–6. 27. Tutt R, Bradley A, Begley C, Thibos LN. Optical and visual impact of tear break-up in human eyes. Invest Ophthalmol Vis Sci. 2000;41:4117–23. 28. Lemp MA. Advances in understanding and managing dry eye disease. Am J Ophthalmology. 2008;146:350–6. 29. Pflugfelder SC. Antiinflammatory therapy for dry eye. Am J Ophthalmol. 2004;137(2):337–42. 30. Zinovkin RA, Romaschenko VP, Galkin II, et al. Role of mitochondrial reactive oxygen species in age-related inflammatory activation of endothelium. Aging (Albany NY). 2014;6(8):661–74
REFERENCES 1. Beckman, K. B., and Ames, B. N. (1998) The free radical theory of aging matures, Physiol. Rev., 78, 547581. 2. Skulachev, V. P. (2005) How to clean the dirtiest place in the cell: cationic antioxidants as intramitochondrial ROS scavengers, IUBMB Life, 57, 305310. 3. Skulachev, V. P. (2006) Bioenergetic aspects of apoptosis, necrosis and mitoptosis, Apoptosis, 11, 473485. 4. Jarrett, S. G., and Boulton, M. E. (2012) Consequences of oxidative stress in agerelated macular degeneration, Mol. Aspects Med., 33, 399417. 5. Panfoli, I. (2012) Beneficial effect of antioxidants in retinopathies: a new hypothesis, Med. Hypothesis Discov. Innov. Ophthalmol., 1, 7679. 6. Newell, F. W. (1992) Ophthalmology Principles and Concepts (Mosby, C. V., ed.) 7th Edn., St. Louis. 7. Winkler, B. S., Boulton, M. E., Gottsch, J. D., and Sternberg, P. (1999) Oxidative damage and agerelated macular degeneration, Mol. Vis., 5, 32. 8. Emerit, J., Edeas, M., and Bricaire, F. (2004) Neurodegenerative diseases and oxidative stress, Biomed. Pharmacother., 58, 3946. 9. Roth, F., Bindewald, A., and Holz, F. G. (2004) Key pathophysiological pathways in agerelated macular dis ease, Graefes Arch. Clin. Exp. Ophthalmol., 242, 710716.
Kopitz, J., Holz, F. G., Kaemmerer, E., and Schutt, F. (2004) Lipids and lipid peroxidation products in the patho genesis of agerelated macular degeneration, Biochimie, 86, 825831. 11. Tanito, M., Nishiyama, A., Tanaka, T., Masutani, H., Nakamura, H., Yodoi, J., and Ohira, A. (2002) Change of redox status and modulation by thiol replenishment in reti nal photooxidative damage, Invest. Ophthalmol. Vis. Sci., 43, 23922400. 12. Neroev, V. V., Archipova, M. M., Bakeeva, L. E., Fursova, A., Grigorian, E. N., Grishanova, A. Y., Iomdina, E. N., Ivashchenko, Zh. N., Katargina, L. A., Khoroshilova Maslova, I. P., Kilina, O. V., Kolosova, N. G., Kopenkin, E. P., Korshunov, S. S., Kovaleva, N. A., Novikova, Y. P., Philippov, P. P., Pilipenko, D. I., Robustova, O. V., Saprunova, V. B., Senin, I. I., Skulachev, M. V., Sotnikova, L. F., Stefanova, N. A., Tikhomirova, N. K., Tsapenko, I. V., Shchipanova, A. I., Zinovkin, R. A., and Skulachev, V. P. (2008) Mitochondriatargeted plastoquinone derivatives as tools to interrupt execution of the aging program. 4. Age related eye disease. SkQ1 returns vision to blind animals, Biochemistry (Moscow), 73, 13171328. 13. Antonenko, Y. N., Avetisyan, A. V., Bakeeva, L. E., Chernyak, B. V., Chertkov, V. A., Domnina, L. V., Ivanova, O. Y., Izyumov, D. S., Khailova, L. S., Klishin, S. S., Korshunova, G. A., Lyamzaev, K. G., Muntyan, M. S., Nepryakhina, O. K., Pashkovskaya, A. A., Pletjushkina, O. Y., Pustovidko, A. V., Roginsky, V. A., Rokitskaya, T. I., Ruuge, E. K., Saprunova, V. B., Severina, I. I., Simonyan, R. A., Skulachev, I. V., Skulachev, M. V., Sumbatyan, N. V., Sviryaeva, I. V., Tashlitsky, V. N., Vassiliev, J. M., Vyssokikh, M. Y., Yaguzhinsky, L. S., Zamyatnin, A. A., and Skulachev, V. P. (2008) Mitochondriatargeted plasto quinone derivatives as tools to interrupt execution of the aging program. 1. Cationic plastoquinone derivatives: syn thesis and in vitro studies, Biochemistry (Moscow), 73, 1273 1287. 14. Agapova, L. S., Chernyak, B. V., Domnina, L. V., Dugina, V. B., Efimenko, A. Y., Fetisova, E. K., Ivanova, O. Y., Kalinina, N. I., Khromova, N. V., Kopnin, B. P., Kopnin, P. B., Korotetskaya, M. V., Lichinitser, M. R., Lukashev, A. L., Pletjushkina, O. Y., Popova, E. N., Skulachev, M. V., Shagieva, G. S., Stepanova, E. V., Titova, E. V., Tkachuk, V. A., Vasiliev, J. M., and Skulachev, V. P. (2008) Mitochondriatargeted plastoquinone derivatives as tools to interrupt execution of the aging program. 3. Inhibitory effect of SkQ1 on tumor development from p53deficient cells, Biochemistry (Moscow), 73, 13001316. 15. Grigoryan, E. N., Novikova, Y. P., Gancharova, O. S., Kilina, O. V., and Philippov, P. P. (2012) New antioxidant SkQ1 is an effective protector of rat eye retinal pigment epithelium and choroid under conditions of longterm organotypic cultivation, Adv. Aging Res., 1, 3137. 16. Grigoryan, E., Novikova, Y., Kilina, O., and Philippov, P. (2013) New antioxidant SkQ1 is an effective protector of rat neural retina under conditions of longterm organotypic cultivation, Adv. Aging Res., 2, 6571. 17. Noell, W. K., Walker, V. S., Kang, B. S., and Berman, S. (1966) Retinal damage by light in rats, Invest. Ophthalmol., 5, 450473. 18. Stone, J., Maslim, J., ValterKocsi, K., Mervin, K., Bowers, F., Chu, Y., Barnett, N., Provis, J., Lewis, G., Fisher, S. K., Bisti, S., Gargini, C., Cervetto, L., Merin, S., and Peer, J. (1999) Mechanisms of photoreceptor death and survival in mammalian retina, Prog. Retin. Eye Res., 18, 689735. 19. Komarek, V., Gembardt, C., Krinke, A.L., Mahrous, T., and Schaetti, P. (2000) Synopsis of the organ anatomy, in The Laboratory Rat (Krinke, G., ed.) Academic Press, San Diego, pp. 283319. 20. Wasowicz, M., Morice, C., Ferrari, P., Callebert, J., and VersauxBotteri, C. (2002) Longterm effects of light dam age on the retina of albino and pigmented rats, Invest. Ophthalmol. Vis. Sci., 43, 813820. 21. Bennett, M. H., Dyer, R. F., and Dunn, J. D. (1972) Light induced retinal degeneration: effect upon lightdark dis crimination, Exp. Neurol., 34, 434445. 22. Williams, R. A., Howard, A. G., and Williams, T. P. (1985) Retinal injury on pigmented and albino rats exposed to low intensive cyclic light after unique mydriatic influence, Curr. Eye Res., 4, 97102. 23. Williams, T. P., and Howell, W. L. (1983) Action spectrum of retinal lightdamage in albino rats, Invest. Ophthalmol. Vis. Sci., 24, 285287. 24. Marc, R. E., Jones, B. W., Watt, C. B., VazquezChona, F., Vaughan, D. K., and Organisciak, D. T. (2008) Extreme retinal remodeling triggered by light damage: implications for age related macular degeneration, Mol. Vis., 14, 782 806. 25. Abler, A. S., Chang, C. J., Ful, J., Tso, M. O., and Lam, T. T. (1996) Photic injury triggers apoptosis of photoreceptor cells, Res. Commun. Mol. Pathol. Pharmacol., 92, 177189. 26. Lin, Y., Jones, B. W., Liu, A., VazquezChona, F. R., Lauritzen, J. S., Ferrell, W. D., and Marc, R. E. (2012) Rapid glutamate receptor 2 trafficking during retinal degeneration, Mol. Neurodegener., 7, 7. 27. Kuwabara, T., and Gorn, R. A. (1968) Retinal damage by visible light. An electron microscopic study, Arch. Ophthalmol., 79, 6978. 28. Schmidt, R. E., and Zuclich, J. A. (1980) Retinal lesions due to ultraviolet laser exposure, Invest. Ophthalmol. Vis. Sci., 19, 11661175. 29. De Vera Mudry, M. C., Kronenberg, S., Komatsu, S., and Aguirre, G. D. (2013) Blinded by the light: retinal photo toxicity in the context of safety studies, Toxicol. Pathol., 41, 813825. 30. Hafezi, F., Marti, A., Munz, K., and Reme, C. E. (1997) Lightinduced apoptosis: differential timing in the retina and pigment epithelium, Exp. Eye Res., 64, 963970. 31. Wenzel, A., Grimm, C., Samardzija, M., and Reme, C. E. (2005) Molecular mechanisms of lightinduced photore ceptor apoptosis and neuroprotection for retinal degenera tion, Prog. Retin. Eye Res., 24, 275306. 32. Costa, B. L., Fawcett, R., Li, G.Y., Safa, R., and Osborne, N. N. (2008) Orally administered epigallocatechin gallate attenuates lightinduced photoreceptor damage, Brain Res. Bull., 76, 412423. 33. Mandal, M. N. A., Patlolla, J. M. R., Zheng, L., Agbaga, M.P., Tran, J.T. A., Wicker, L., KasusJacobi, A., Elliott, M. H., Rao, C. V., and Anderson, R. E. (2009) Curcumin protects retinal cells from light and oxidant stressinduced cell death, Free Radic. Biol. Med., 46, 672679. 34. Tanito, M., Li, F., Elliott, M. H., Dittmar, M., and Anderson, R. E. (2007) Protective effect of TEMPOL
derivatives against lightinduced retinal damage in rats, Invest. Ophthalmol. Vis. Sci., 48, 19001905. 35. Lewis, G. P., Guerin, C. J., Anderson, D. H., Matsumoto, B., and Fisher, S. K. (1994) Rapid changes in the expres sion of glial cell proteins caused by experimental retinal detachment, Am. J. Ophthalmol., 118, 368376. 36. Fisher, S. K., Erickson, P. A., Lewis, G. P., and Anderson, D. H. (1991) Intraretinal proliferation induced by retinal detachment, Invest. Ophthalmol. Vis. Sci., 32, 17391748. 37. Bringmann, A., Pannicke, T., Grosche, J., Francke, M., Wiedemann, P., Skatchkov, S. N., Osborne, N. N., and Reichenbach, A. (2006) Muller cells in the healthy and dis eased retina, Prog. Retin. Eye Res., 25, 397424. 38. Jadhav, A. P., Roesch, K., and Cepko, C. L. (2009) Development and neurogenic potential of Muller glial cells in the vertebrate retina, Prog. Retin. Eye Res., 28, 249262. 39. Cachafeiro, M., Bemelmans, A. P., Samardzija, M., Afanasieva, T., Pournaras, J. A., Grimm, C., Kostic, C., Philippe, S., Wenzel, A., and Arsenijevic, Y. (2013) Hyperactivation of retina by light in mice leads to photore ceptor cell death mediated by VEGF and retinal pigment epithelium permeability, Cell Death Dis., 4, e781. 40. Greenwood, J. (1992) The bloodretinal barrier in experi mental autoimmune uveoretinitis (EAU): a review, Curr. Eye Res, 11 (Suppl.), 2532. 41. Perche, O., Doly, M., and RanchonCole, I. (2007) Caspasedependent apoptosis in lightinduced retinal degeneration, Invest. Ophthalmol. Vis. Sci., 48, 27532759. 42. Antonenko, Y. N., Roginsky, V. A., Pashkovskaya, A. A., Rokitskaya, T. I., Kotova, E. A., Zaspa, A. A., Chernyak, B. V., and Skulachev, V. P. (2008) Protective effects of mito chondriatargeted antioxidant SkQ in aqueous and lipid membrane environments, J. Membr. Biol., 222, 141149. 43. Chernyak, B. V., Izyumov, D. S., Lyamzaev, K. G., Pashkovskaya, A. A., Pletjushkina, O. Y., Antonenko, Y. N., Sakharov, D. V., Wirtz, K. W. A., and Skulachev, V. P. (2006) Production of reactive oxygen species in mitochon dria of HeLa cells under oxidative stress, Biochim. Biophys. Acta, 1757, 525534. 44. Gordon, W. C., Casey, D. M., Lukiw, W. J., and Bazan, N. G. (2002) DNA damage and repair in lightinduced pho toreceptor degeneration, Invest. Ophthalmol. Vis. Sci., 43, 35113521. 45. Xia, X., Li, Y., Huang, D., Wang, Z., Luo, L., Song, Y., Zhao, L., and Wen, R. (2011) Oncostatin M protects rod and cone photoreceptors and promotes regeneration of cone outer segment in a rat model of retinal degeneration, PLoS One, 6, e18282. 46. Wen, R., Tao, W., Luo, L., Huang, D., Kauper, K., Stabila, P., LaVail, M. M., Laties, A. M., and Li, Y. (2012) Regeneration of cone outer segments induced by CNTF, Adv. Exp. Med. Biol., 723, 9399. 47. Youssef, P. N., Sheibani, N., and Albert, D. M. (2011) Retinal light toxicity, Eye, 25, 114. 48. Forooghian, F., Stetson, P. F., Gross, N. E., and Meyerle, C. B. (2010) Quantitative assessment of photoreceptor recovery in atypical multiple evanescent white dot syn drome, Ophthalm. Surg. Lasers Imag., 41 (Suppl.), 7780. 49. Tanito, M., Kaidzu, S., Ohira, A., and Anderson, R. E. (2008) Topography of retinal damage in lightexposed albi no rats, Exp. Eye Res., 87, 292295. 50. Organisciak, D. T., Darrow, R. M., Rapp, C. M., Smuts, J. P., Armstrong, D. W., and Lang, J. C. (2013) Prevention of retinal light damage by zinc oxide combined with rosemary extract, Mol. Vis., 19, 14331445. 51. Ojino, K., Shimazawa, M., Ohno, Y., Otsuka, T., Tsuruma, K., and Hara, H. (2014) Protective effect of SUN N8075, a free radical scavenger, against excessive lightinduced reti nal damage in mice, Biol. Pharm. Bull., 37, 424430. 52. Arnault, E., Barrau, C., Nanteau, C., Gondouin, P., Bigot, K., Vienot, F., Gutman, E., Fontaine, V., Villette, T., CohenTannoudji, D., Sahel, J.A., and Picaud, S. (2013) Phototoxic action spectrum on a retinal pigment epitheli um model of agerelated macular degeneration exposed to sunlight normalized conditions, PLoS One, 8, e71398. 53. Kernt, M., Thiele, S., Neubauer, A. S., Koenig, S., Hirneiss, C., Haritoglou, C., Ulbig, M. W., and Kampik, A. (2012) Inhibitory activity of ranibizumab, sorafenib, and pazopanib on lightinduced overexpression of platelet derived growth factor and vascular endothelial growth fac tor A and the vascular endothelial growth factor A receptors 1 and 2 and neuropilin 1 and 2, Retina, 32, 16521663. 54. Saprunova, V. B., Pilipenko, D. I., Alexeevsky, A. V., Fursova, A. Z., Kolosova, N. G., and Bakeeva, L. E. (2010) Lipofuscin granule dynamics during development of age related macular degeneration, Biochemistry (Moscow), 75, 130138. 55. Markovets, A. M., Fursova, A. Z., and Kolosova, N. G. (2011) Therapeutic action of the mitochondriatargeted antioxidant SkQ1 on retinopathy in OXYS rats linked with improvement of VEGF and PEDF gene expression, PLoS One, 6, e21682.
References Andreev-Andrievskiy AA, Kolosova NG, Stefanova NA, Lovat MV, Egorov MV, Manskikh VN, Zinovkin RA, Galkin II, Prikhodko AS, Skulachev MV, Lukashev AN (2016) Efficacy of mitochondrial antioxidant plastoquinonyl-decyl-triphenylphosphonium bromide (SkQ1) in the rat model of autoimmune arthritis. Oxidative Med Cell Longev 2016:8703645 Antonenko YN, Avetisyan AV, Bakeeva LE, Chernyak BV, Chertkov VA, Domnina LV, Ivanova OY, Izyumov DS, Khailova LS, Klishin SS, Korshunova GA, Lyamzaev KG, Muntyan MS, Nepryakhina OK, Pashkovskaya AA, Pletjushkina OY, Pustovidko AV, Roginsky VA, Rokitskaya TI, Ruuge EK, Saprunova VB, Severina II, Simonyan RA, Skulachev IV, Skulachev MV, Sumbatyan NV, Sviryaeva IV, Tashlitsky VN, Vassiliev JM, Vyssokikh MY, Yaguzhinsky LS, Zamyatnin AA Jr, Skulachev VP (2008) Mitochondria-targeted plastoquinone derivatives as tools to interrupt execution of the aging program. 1. Cationic plastoquinone derivatives: synthesis and in vitro studies. Biochemistry (Mosc) 73:1273–1287 Bakeeva LE, Grinius LL, Jasaitis AA, Kuliene VV, Levitsky DO, Liberman EA, Severina II, Skulachev VP (1970) Conversion of biomembrane-produced energy into electric form. II. Intact mitochondria. Biochim Biophys Acta 216:13–21 Bakeeva LE, Barskov IV, Egorov MV, Isaev NK, Kapelko VI, Kazachenko AV, Kirpatovsky VI, Kozlovsky SV, Lakomkin VL, Levina SB, Pisarenko OI, Plotnikov EY, Saprunova VB, Serebryakova LI, Skulachev MV, Stelmashook EV, Studneva IM, Tskitishvili OV, Vasilyeva AK, Victorov IV, Zorov DB, Skulachev VP (2008) Mitochondria-targeted plastoquinone derivatives as tools to interrupt execution of the aging program. 2. Treatment of some ROSand age-related diseases (heart arrhythmia, heart infarctions, kidney ischemia, and stroke). Biochemistry (Mosc) 73:1288–1299 Bakeeva LE, Eldarov CM, Vangely IM, Kolosova NG, Vays VB (2016) Mitochondria-targeted antioxidant SkQ1 reduces age-related alterations in the ultrastructure of the lacrimal gland. Oncotarget 7:80208–80222 Beatty S, Koh H, Phil M, Henson D, Boulton M (2000) The role of oxidative stress in the pathogenesis of age-related macular degeneration. Surv Ophthalmol 45:115–134 Brzheskiy VV, Efimova EL, Vorontsova TN, Alekseev VN, Gusarevich OG, Shaidurova KN, Ryabtseva AA, Andryukhina OM, Kamenskikh TG, Sumarokova ES, Miljudin ES, Egorov EA, Lebedev OI, Surov AV, Korol AR, Nasinnyk IO, Bezditko PA, Muzhychuk OP, Vygodin VA, Yani EV, Savchenko AY, Karger EM, Fedorkin ON, Mironov AN, Ostapenko V, Popeko NA, Skulachev VP, Skulachev MV (2015) Results of a multicenter, randomized, double-masked, placebo-controlled clinical study of the efficacy and safety of visomitin eye drops in patients with dry eye syndrome. Adv Ther 32:1263–1279 Erichev VP, Kozlova IV, Reshchikova VS, Alekseev VN, Levko MA, Zamyatnin AAJ, Gudkova EY, Kovaleva NA, Vygodin VA, Fedorkin ON, Ostapenko V, Senin II, Savchenko AY, Popeko NA, Skulachev VP, Skulachev MV (2016) Efficacy and safety of Visomitin® eye drops, in patients with age-related cataract: a randomized, double-blind, placebo-controlled clinical study. Nat.J.Glaucoma(Rus.) 1:61–69 Feniouk BA, Skulachev VP (2018) Studies on mitochondria directed plastoquinones. In: Oliveira PJ (ed) Mitochondrial biology and experimental therapeutics. Springer, New York Galkin II, Pletjushkina OY, Zinovkin RA, Zakharova VV, Chernyak BV, Popova EN (2016) Mitochondria-targeted antioxidant SkQR1 reduces TNF-induced endothelial permeability in vitro. Biochemistry (Mosc) 81:1188–1197 Green DE (1974) The electromechanochemical model for energy coupling in mitochondria. Biochim Biophys Acta 346:27–78 Iomdina EN, Khoroshilova-Maslova IP, Robustova OV, Averina OA, Kovaleva NA, Aliev G, Reddy VP, Zamyatnin AA Jr, Skulachev MV, Senin II, Skulachev VP (2015) Mitochondriatargeted antioxidant SkQ1 reverses glaucomatous lesions in rabbits. Front Biosci (Landmark Ed) 20:892–901 Isaev NK, Novikova SV, Stelmashook EV, Barskov IV, Silachev DN, Khaspekov LG, Skulachev VP, Zorov DB (2012) Mitochondria-targeted plastoquinone antioxidant SkQR1 decreases trauma-induced neurological deficit in rat. Biochemistry (Mosc) 77:996–999 James AM, Cocheme HM, Smith RA, Murphy MP (2005) Interactions of mitochondria-targeted and untargeted ubiquinones with the mitochondrial respiratory chain and reactive oxygen species. Implications for the use of exogenous ubiquinones as therapies and experimental tools. J Biol Chem 280:21295–21312 Jiang Y, Liu C, Lei B, Xu X, Lu B (2017) Mitochondria-targeted antioxidant SkQ1 improves spermatogenesis in Immp2l mutant mice. Andrologia. https://doi.org/10.1111/and.12848 Kanda A, Chen W, Othman M, Branham KE, Brooks M, Khanna R, He S, Lyons R, Abecasis GR, Swaroop A (2007) A variant of mitochondrial protein LOC387715/ARMS2, not HTRA1, is strongly associated with age-related macular degeneration. Proc Natl Acad Sci U S A 104:16227–16232 Kapay NA, Isaev NK, Stelmashook EV, Popova OV, Zorov DB, Skrebitsky VG, Skulachev VP (2011) In vivo injected mitochondria-targeted plastoquinone antioxidant SkQR1 prevents beta-amyloid-induced decay of long-term potentiation in rat hippocampal slices. Biochemistry (Mosc) 76:1367–1370 Kapay NA, Popova OV, Isaev NK, Stelmashook EV, Kondratenko RV, Zorov DB, Skrebitsky VG, Skulachev VP (2013) Mitochondria-targeted plastoquinone antioxidant SkQ1 prevents amyloid-beta-induced impairment of long-term potentiation in rat hippocampal slices. J Alzheimers Dis 36:377–383 Kelso GF, Porteous CM, Coulter CV, Hughes G, Porteous WK, Ledgerwood EC, Smith RA, Murphy MP (2001) Selective targeting of a redox-active ubiquinone to mitochondria within cells: antioxidant and antiapoptotic properties. J Biol Chem 276:4588–4596 Kolosova NG, Shcheglova TV, Sergeeva SV, Loskutova LV (2006) Long-term antioxidant supplementation attenuates oxidative stress markers and cognitive deficits in senescent-accelerated OXYS rats. Neurobiol Aging 27:1289–1297 Kolosova NG, Tyumentsev MA, Muraleva NA, Kiseleva E, Vitovtov AO, Stefanova NA (2017) Antioxidant SkQ1 alleviates signs of Alzheimer’s disease-like pathology in old OXYS rats by reversing mitochondrial deterioration. Curr Alzheimer Res 14(12):1283–1292 Liberman EA, Topaly VP, Tsofina LM, Jasaitis AA, Skulachev VP (1969) Mechanism of coupling of oxidative phosphorylation and the membrane potential of mitochondria. Nature 222:1076–1078 Manskikh VN, Gancharova OS, Nikiforova AI, Krasilshchikova MS, Shabalina IG, Egorov MV, Karger EM, Milanovsky GE, Galkin II, Skulachev VP, Zinovkin RA (2015) Age-associated murine cardiac lesions are attenuated by the mitochondria-targeted antioxidant SkQ1. Histol Histopathol 30:353–360 SkQ1: The Road from Laboratory Bench to the Market 644 Markovets AM, Fursova AZ, Kolosova NG (2011) Therapeutic action of the mitochondria-targeted antioxidant SkQ1 on retinopathy in OXYS rats linked with improvement of VEGF and PEDF gene expression. PLoS One 6:e21682 Muraleva NA, Kozhevnikova OS, Zhdankina AA, Stefanova NA, Karamysheva TV, Fursova AZ, Kolosova NG (2014) The mitochondria-targeted antioxidant SkQ1 restores alphaB-crystallin expression and protects against AMD-like retinopathy in OXYS rats. Cell Cycle 13:3499–3505 Neroev VV, Archipova MM, Bakeeva LE, Fursova AZ, Grigorian EN, Grishanova AY, Iomdina EN, Ivashchenko ZN, Katargina LA, Khoroshilova-Maslova IP, Kilina OV, Kolosova NG, Kopenkin EP, Korshunov SS, Kovaleva NA, Novikova YP, Philippov PP, Pilipenko DI, Robustova OV, Saprunova VB, Senin II, Skulachev MV, Sotnikova LF, Stefanova NA, Tikhomirova NK, Tsapenko IV, Shchipanova AI, Zinovkin RA, Skulachev VP (2008) Mitochondria-targeted plastoquinone derivatives as tools to interrupt execution of the aging program. 4. Age-related eye disease. SkQ1 returns vision to blind animals. Biochemistry (Mosc) 73:1317–1328 Ousler GW, Gomes PJ, Welch D, Abelson MB (2005) Methodologies for the study of ocular surface disease. Ocul Surf 3:143–154 Pasyukova EG, Feniouk BA, Skulachev VP (2017) Mitochondria-targeted rechargeable antioxidants as potential anti-aging drugs. In: Vaiserman A (ed) Anti-aging drugs: from basic research to clinical practice. Royal Society of Chemistry, London Perepechaeva ML, Grishanova AY, Rudnitskaya EA, Kolosova NG (2014) The mitochondriatargeted antioxidant SkQ1 downregulates aryl hydrocarbon receptor-dependent genes in the retina of OXYS rats with AMD-like retinopathy. J Ophthalmol 2014:530943 Petrov A, Perekhvatova N, Skulachev M, Stein L, Ousler G (2016) SkQ1 ophthalmic solution for dry eye treatment: results of a phase 2 safety and efficacy clinical study in the environment and during challenge in the controlled adverse environment model. Adv Ther 33:96–115 Plotnikov EY, Chupyrkina AA, Jankauskas SS, Pevzner IB, Silachev DN, Skulachev VP, Zorov DB (2011) Mechanisms of nephroprotective effect of mitochondria-targeted antioxidants under rhabdomyolysis and ischemia/reperfusion. Biochim Biophys Acta 1812:77–86 Plotnikov EY, Morosanova MA, Pevzner IB, Zorova LD, Manskikh VN, Pulkova NV, Galkina SI, Skulachev VP, Zorov DB (2013) Protective effect of mitochondria-targeted antioxidants in an acute bacterial infection. Proc Natl Acad Sci U S A 110:E3100–E3108 Rumyantseva YV, Ryabchikova EI, Fursova AZ, Kolosova NG (2015) Ameliorative effects of SkQ1 eye drops on cataractogenesis in senescence-accelerated OXYS rats. Graefes Arch Clin Exp Ophthalmol 253:237–248 Saprunova VB, Lelekova MA, Kolosova NG, Bakeeva LE (2012) SkQ1 slows development of agedependent destructive processes in retina and vascular layer of eyes of wistar and OXYS rats. Biochemistry (Mosc) 77:648–658 Sergeeva S, Bagryanskaya E, Korbolina E, Kolosova N (2006) Development of behavioural dysfunctions in accelerated-senescence OXYS rats is associated with early postnatal alterations in brain phosphate metabolism. Exp Gerontol 41:141–150 Shabalina IG, Vyssokikh MY, Gibanova N, Csikasz RI, Edgar D, Hallden-Waldemarson A, Rozhdestvenskaya Z, Bakeeva LE, Vays VB, Pustovidko AV, Skulachev MV, Cannon B, Skulachev VP, Nedergaard J (2017) Improved health-span and lifespan in mtDNA mutator mice treated with the mitochondrially targeted antioxidant SkQ1. Aging (Albany NY) 9:315–339 Skulachev VP, Anisimov VN, Antonenko YN, Bakeeva LE, Chernyak BV, Erichev VP, Filenko OF, Kalinina NI, Kapelko VI, Kolosova NG, Kopnin BP, Korshunova GA, Lichinitser MR, Obukhova LA, Pasyukova EG, Pisarenko OI, Roginsky VA, Ruuge EK, Senin II, Severina II, Skulachev MV, Spivak IM, Tashlitsky VN, Tkachuk VA, Vyssokikh MY, Yaguzhinsky LS, Zorov DB (2009) An attempt to prevent senescence: a mitochondrial approach. Biochim Biophys Acta 1787:437–461 Skulachev MV, Antonenko YN, Anisimov VN, Chernyak BV, Cherepanov DA, Chistyakov VA, Egorov MV, Kolosova NG, Korshunova GA, Lyamzaev KG, Plotnikov EY, Roginsky VA, Savchenko AY, Severina II, Severin FF, Shkurat TP, Tashlitsky VN, Shidlovsky KM, Vyssokikh MY, Zamyatnin AA Jr, Zorov DB, Skulachev VP (2011) Mitochondrial-targeted plastoquinone derivatives. Effect on senescence and acute age-related pathologies. Curr Drug Targets 12:800–826 Stefanova NA, Muraleva NA, Maksimova KY, Rudnitskaya EA, Kiseleva E, Telegina DV, Kolosova NG (2016) An antioxidant specifically targeting mitochondria delays progression of Alzheimer’s disease-like pathology. Aging (Albany NY) 8:2713–2733 Yanshole LV, Yanshole VV, Snytnikova OA, Fursova A, Kolosova NG, Tsentalovich YP, Sagdeev RZ (2015) Effect of SkQ1 eye drops on the rat lens metabolomic composition and the chaperone activity of alpha-crystallin. Dokl Biochem Biophys 464:341–345 Zakharova VV, Pletjushkina OY, Galkin II, Zinovkin RA, Chernyak BV, Krysko DV, Bachert C, Krysko O, Skulachev VP, Popova EN (2017) Low concentration of uncouplers of oxidative phosphorylation decreases the TNF-induced endothelial permeability and lethality in mice. Biochim Biophys Acta 1863:968–977 Zhang YB, Meng YH, Chang S, Zhang RY, Shi C (2016) High fructose causes cardiac hypertrophy via mitochondrial signaling pathway. Am J Transl Res 8:4869–4880 Zinovkin RA, Romaschenko VP, Galkin II, Zakharova VV, Pletjushkina OY, Chernyak BV, Popova EN (2014) Role of mitochondrial reactive oxygen species in age-related inflammatory activation of endothelium. Aging (Albany NY) 6:661–674 REFERENCES 1. V. P. Skulachev, Biokhimiya, 72, 1700–1714 (2007). 2. V. M. Skulachev, Y. N. Antonenko, V. N. Anisimov, et al., Curr. Drug Targets, 12(6), 800–826 (2011). 3. E. V. Yani, L. A. Katargina, N. B. Chesnokova, et al., Prakticheskaya Meditsina, 04 (12); Oftal’mologiya, 1, 134–137 (2012). 4. L. T. Arkhipova, M. M. Arkhipova, L. E. Bakeeva, et al., Biokhimiya, 73(12), 1641–1654 (2008). 5. V. B. Saprunova, D. I. Pilipenko, A. V. Alekseevskii, et al., Biokhimiya, 75(2), 158–168 (2010). 6. N. A. Stefanova, A. Zh. Fursova, N. G. Kolosova, J. Alzheimer’s Dis., 21(2), 479–491 (2010). 7. A. M. Markovets, A. Z. Fursova, N. G. Kolosova, PLoS One, 6(7), 5 (2011). 8. E. N. Grigoryan, Y. P. Novikova, O. S. Gancharova, et al., Adv. Aging Res., 1(2), 31–37 (2012). 9. K. C. Waterman, R. C. Adami, Int. J. Pharm., 293, 101–125 (2005). 10. A. A. Matyushin, D. A. Tsarev, M. A. Grigorenko, et al., Farmatsiya, 5, 23–29 (2008). 11. Table of Typical Illuminances. URL: http: // broyhill.edublogs.org / 2009 / 08 / 09 / lux (accessed Oct. 17, 2012).
REFERENCES 1. Skulachev VP. Cationic antioxidants as a powerful tool against mitochondrial oxidative stress. Biochem Biophys Res Commun. 2013;441:275–279. 2. Grundlingh J, Dargan PI, El-Zanfaly M, Wood DM. 2,4-Dinitrophenol (DNP): a weight loss agent with significant acute toxicity and risk of death. J Med Toxicol. 2011;7:205–212. 3. Rosenberg LE, Fenton WA, Horwich AL, Kalousek F, Kraus JP. Targeting of nuclearencoded proteins to the mitochondrial matrix: implications for human genetic defects. Ann N Y Acad Sci. 1986;488:99–108. 4. Sakhrani NM, Padh H. Organelle targeting: third level of drug targeting. Drug Des Devel Ther. 2013;7:585–599. 5. Liberman EA, Topaly VP, Tsofina LM, Jasaitis AA, Skulachev VP. Mechanism of coupling of oxidative phosphorylation and the membrane potential of mitochondria. Nature. 1969;222:1076–1078. 6. Bakeeva LE, Grinius LL, Jasaitis AA, et al. Conversion of biomembrane-produced energy into electric form. II. Intact mitochondria. Biochim Biophys Acta. 1970;216:13–21. 7. Zhao K, Zhao GM, Wu D, et al. Cell-permeable peptide antioxidants targeted to inner mitochondrial membrane inhibit mitochondrial swelling, oxidative cell death, and reperfusion injury. J Biol Chem. 2004;279:34682–34690. 8. Zorov DB, Filburn CR, Klotz LO, Zweier JL, Sollott SJ. Reactive oxygen species (ROS)-induced ROS release: a new phenomenon accompanying induction of the mitochondrial permeability transition in cardiac myocytes. J Exp Med. 2000;192:1001–1014. 9. Kelso GF, Porteous CM, Coulter CV, et al. Selective targeting of a redox-active ubiquinone to mitochondria within cells: antioxidant and antiapoptotic properties. J Biol Chem. 2001;276:4588–4596. 10. Antonenko YN, Avetisyan AV, Bakeeva LE, et al. Mitochondria-targeted plastoquinone derivatives as tools to interrupt execution of the aging program. 1. Cationic plastoquinone derivatives: synthesis and in vitro studies. Biochemistry (Mosc). 2008;73:1273–1287. 11. Adlam VJ, Harrison JC, Porteous CM, et al. Targeting an antioxidant to mitochondria decreases cardiac ischemia-reperfusion injury. FASEB J. 2005;19:1088–1095. 12. Plotnikov EY, Silachev DN, Chupyrkina AA, et al. New-generation Skulachev ions exhibiting nephroprotective and neuroprotective properties. Biochemistry (Mosc). 2010;75:145–150. 13. Bakeeva LE, Barskov IV, Egorov MV, et al. Mitochondria-targeted plastoquinone derivatives as tools to interrupt execution of the aging program. 2. Treatment of some ROS- and age-related diseases (heart arrhythmia, heart infarctions, kidney ischemia, and stroke). Biochemistry (Mosc). 2008;73:1288–1299. 14. Skulachev VP, Anisimov VN, Antonenko YN, et al. An attempt to prevent senescence: a mitochondrial approach. Biochim Biophys Acta. 2009;1787:437–461. 15. Skulachev VP, Longo VD. Aging as a mitochondria-mediated atavistic program: can aging be switched off? Ann N Y Acad Sci. 2005;1057:145–164. 16. Anisimov VN, Bakeeva LE, Egormin PA, et al. Mitochondria-targeted plastoquinone derivatives as tools to interrupt execution of the aging program. 5. SkQ1 prolongs lifespan and prevents development of traits of senescence. Biochemistry (Mosc). 2008;73:1329–1342. 17. Krementsova AV, Roshina NV, Tsybul’ko EA, Rybina OY, Symonenko AV, Pasyukova EG. Reproducible effects of the mitochondria-targeted plastoquinone derivative SkQ1 on Drosophila melanogaster lifespan under different experimental scenarios. Biogerontology. 2012;13:595–607. 18. Skulachev MV, Antonenko YN, Anisimov VN, et al. Mitochondrial-targeted plastoquinone derivatives. Effect on senescence and acute age-related pathologies. Curr Drug Targets. 2011;12:800–826.
Anisimov VN, Egorov MV, Krasilshchikova MS, et al. Effects of the mitochondria-targeted antioxidant SkQ1 on lifespan of rodents. Aging (Albany NY). 2011;3:1110–1119. 20. Kolosova NG, Stefanova NA, Muraleva NA, Skulachev VP. The mitochondria-targeted antioxidant SkQ1 but not N-acetylcysteine reverses aging-related biomarkers in rats. Aging (Albany NY). 2012;4:686–694. 21. Neroev VV, Archipova MM, Bakeeva LE, et al. Mitochondria-targeted plastoquinone derivatives as tools to interrupt execution of the aging program. 4. Age-related eye disease. SkQ1 returns vision to blind animals. Biochemistry (Mosc). 2008;73:1317–1328. 22. Chacko BK, Srivastava A, Johnson MS, et al. Mitochondria-targeted ubiquinone (MitoQ) decreases ethanol-dependent micro and macro hepatosteatosis. Hepatology. 2011;54:153–163. 23. Lowes DA, Thottakam BM, Webster NR, Murphy MP, Galley HF. The mitochondriatargeted antioxidant MitoQ protects against organ damage in a lipopolysaccharidepeptidoglycan model of sepsis. Free Radic Biol Med. 2008;45:1559–1565. 24. Gane EJ, Weilert F, Orr DW, et al. The mitochondria-targeted anti-oxidant mitoquinone decreases liver damage in a phase II study of hepatitis C patients. Liver Int. 2010;30:1019–1026. 25. Au JS, Pockros PJ. Novel therapeutic approaches for hepatitis C. Clin Pharmacol Ther. 2014;95:78–88. 26. Pelletier M, Lepow TS, Billingham LK, Murphy MP, Siegel RM. New tricks from an old dog: mitochondrial redox signaling in cellular inflammation. Semin Immunol. 2012;24:384–392. 27. Shipounova IN, Svinareva DA, Petrova TV, et al. Reactive oxygen species produced in mitochondria are involved in age-dependent changes of hematopoietic and mesenchymal progenitor cells in mice. A study with the novel mitochondria-targeted antioxidant SkQ1. Mech Ageing Dev. 2010;131:415–421. 28. Yang Y, Karakhanova S, Soltek S, Werner J, Philippov PP, Bazhin AV. In vivo immunoregulatory properties of the novel mitochondria-targeted antioxidant SkQ1. Mol Immunol. 2012;52:19–29. 29. Plotnikov EY, Morosanova MA, Pevzner IB, et al. Protective effect of mitochondriatargeted antioxidants in an acute bacterial infection. Proc Natl Acad Sci U S A. 2013;110:E3100–E3108. 30. Demianenko IA, Vasilieva TV, Domnina LV, et al. Novel mitochondria-targeted antioxidants, “Skulachev-ion” derivatives, accelerate dermal wound healing in animals. Biochemistry (Mosc). 2010;75:274–280. 31. Romashchenko VP, Galkin II, Zakharova VV, et al. Mitochondria-targeted compounds decrease TNF-α-dependent endothelium activation. FEBS J. 2013;280(Suppl 1):246. 32. Tschopp J, Schroder K. NLRP3 inflammasome activation: the convergence of multiple signalling pathways on ROS production? Nat Rev Immunol. 2010;10:210–215. 33. Dashdorj A, Jyothi KR, Lim S, et al. Mitochondria-targeted antioxidant MitoQ ameliorates experimental mouse colitis by suppressing NLRP3 inflammasome-mediated inflammatory cytokines. BMC Med. 2013;11:178. 34. Mao P, Manczak M, Shirendeb UP, Reddy PH. MitoQ, a mitochondria-targeted antioxidant, delays disease progression and alleviates pathogenesis in an experimental autoimmune encephalomyelitis mouse model of multiple sclerosis. Biochim Biophys Acta. 1832;2013:2322–2331. 35. Lowes DA, Webster NR, Murphy MP, Galley HF. Antioxidants that protect mitochondria reduce interleukin-6 and oxidative stress, improve mitochondrial function, and reduce biochemical markers of organ dysfunction in a rat model of acute sepsis. Br J Anaesth. 2013;110:472–480. Over-the-counter drug Drug legal status, Dry eye Medical condition, CVS Pharmacy Retail company, CVS Health Healthcare company, systane eye drops, CVS Photo Topic, Dry eye syndrome Syndrome, Conjunctivitis , Tears Body water, Contact lens, Walgreens, Refresh, Allergy, Antihistamine , Ketotifen , Olopatadine, Lubricant, Artificial tears, Medical prescription, Bausch + Lomb Alaway Antihistamine Eye Drops, SYSTANE , Solution, Tears, Zaditor Antihistamine Eye Drops, Glaucoma, Red eye, Cataract, pataday eye drops, Seattle-Tacoma WA100, Yakima-Pasco-Richland-Kennewick WA97, Spokane WA62, Portland OR, Dry eye syndrome,Breakout, Dry eye syndrome,Breakout, Red,Breakout, Red eye,Breakout, Artificial tears,Breakout, Ophthalmology, Bausch + Lomb LUMIFY, Rohto, Adverse effect , Contact lens, eye drops for dry eyes, best eye drops, dog eye drops, pink eye, pink eye drops, refresh eye drops, pataday eye drops, allergy eye drops, zaditor eye drops, lumify eye drops, stye eye drops, prednisolone eye drops, restasis eye drops, alaway eye drops, steroid eye drops, eye drops for contacts, atropine eye drops, clear eyes eye drops, best dry eye drops, visine eye drops, antihistamine eye drops, preservative free eye drops, Palm Springs , Los Angeles , San Diego , Fresno-Visalia , Monterey-Salinas , Bakersfield , San Francisco-Oakland-San Jose , Santa Barbara-Santa Maria-San Luis Obispo , Sacramento-Stockton-Modesto , Chico-Redding , Yuma AZ-El Centro , Medford-Klamath Falls , Reno NV, Eureka , Reno , Las Vegas , Salt Lake City , , Eye drop, Eye, Drop, Conjunctivitis, Dose, Over-the-counter drug, Dry eye syndrome, Bausch + Lomb LUMIFY, Allergy, Dry eye, Olopatadine, Red eye, Red, Zaditor Antihistamine Eye Drops, SYSTANE, Visual perception, Refresh, Stye, Ketotifen, Visine, Contact lens, Similasan, Tears, Eye infection, , Zaditor Antihistamine Eye Drops,Breakout, SYSTANE,Breakout, Stye,Breakout, Similasan,Breakout, Tears,Breakout, Olopatadine, Over-the-counter drug, Bausch + Lomb LUMIFY, Tucson (Sierra Vista) AZ,100, Phoenix AZ,75, Yuma AZ-El Centro CA,28, Cataract,Breakout, Latanoprost,Breakout, Zaditor Antihistamine Eye Drops,Breakout, Visine,Breakout, SYSTANE,Breakout, Ingredient,Breakout, Can-C,Breakout, Rohto,Breakout, Bausch + Lomb LUMIFY,+450%, Over-the-counter drug,+450%, Ketotifen,+300%, Antihistamine,+190%, Dry eye syndrome,+190%, Steroid,+180%, Stye,+180%, Prednisolone,+140%, Olopatadine,+140%, Dry eye,+120%, Timolol,+90%, Refresh,+50%, Conjunctivitis,+50%, Burn,+40%, Cyclosporine,+40%, Lens,+40%, Glaucoma,+40%, Eyelid,Breakout, Bausch + Lomb LUMIFY,Breakout, Cataract,Breakout, Tears,Breakout, Olopatadine,Breakout, Cataract surgery,Breakout, Stye,Breakout, Over-the-counter drug,+170%, Ketotifen,+80%, Eye,100, Eye drop,90, Drop,75, Dry eye syndrome,8, Contact lens,8, Preservative,6, Over-the-counter drug,6, Allergy,6, Dry eye,6, Eyelid,4, Bausch + Lomb LUMIFY,4, Cataract,4, Ketotifen,4, Tears,4, Olopatadine,2, Cataract surgery,2, Conjunctivitis,2, Stye,2, Red,2, Adverse effect,2, Boise ID,100, Idaho Falls-Pocatello ID,73, Twin Falls ID,72, Spokane WA,18, Great Falls MT,100, Billings, MT,84, Missoula MT,60, Butte-Bozeman MT,45, Glendive MT,, Helena MT,, Minot-Bismarck-Dickinson(Williston) ND,, Cheyenne WY-Scottsbluff NE,100, Billings, MT,16, Rapid City SD,11, Idaho Falls-Pocatello ID,3, Salt Lake City UT,3, Denver CO,2, Casper-Riverton WY,, Grand Junction-Montrose CO,100, Colorado Springs-Pueblo CO,95, Denver CO,85, Albuquerque-Santa Fe NM,100, El Paso TX,17, Amarillo TX,9, Minot-Bismarck-Dickinson(Williston) ND,100, Fargo-Valley City ND,67, Rapid City SD,100, Sioux Falls(Mitchell) SD,62, Lincoln & Hastings-Kearney NE,100, Omaha NE,84, Sioux City IA,15, Cheyenne WY-Scottsbluff NE,12, Sioux Falls(Mitchell) SD,3, Denver CO,<1, North Platte NE,, Topeka KS,100, Wichita-Hutchinson KS,82, Joplin MO-Pittsburg KS,31, Kansas City MO,27, St. Joseph MO,, Oklahoma City OK,100, Tulsa OK,94, Sherman TX-Ada OK,68, Wichita Falls TX & Lawton OK,67, Ft. Smith-Fayetteville-Springdale-Rogers AR,6, Amarillo TX,4, Besifloxacin, Cromoglicic acid, Gatifloxacin,Breakout, Bimatoprost,Breakout, Sulfacetamide,Breakout, Cat\’s eye,Breakout, Eye examination,Breakout, Hydrogen peroxide contact solutions, Beaumont-Port Arthur TX,100, Tyler-Longview(Lufkin & Nacogdoches) TX,87, San Antonio TX,78, Abilene-Sweetwater TX,77, Dallas-Ft. Worth TX,72, Odessa-Midland TX,72, Houston TX,72, El Paso TX,70, Laredo TX,67, Lubbock TX,67, Harlingen-Weslaco-Brownsville-McAllen TX,64, Austin TX,63, Waco-Temple-Bryan TX,60, Corpus Christi TX,55, San Angelo TX,49, Amarillo TX,41, Sherman TX-Ada OK,35, Victoria TX,31, Wichita Falls TX & Lawton OK,24, Shreveport LA,22, Solution, Bimatoprost, Serum, Pharmaceutical drug, Over-the-counter drug, Rochester MN-Mason City IA-Austin MN,100, Minneapolis-St. Paul MN,82, Duluth MN-Superior WI,55, Mankato MN,46, Fargo-Valley City ND,26, La Crosse-Eau Claire WI,3, Moxifloxacin , Cedar Rapids-Waterloo-Iowa City & Dubuque IA,100, Des Moines-Ames IA,76, Davenport IA-Rock Island-Moline IL,60, Sioux City IA,57, Rochester MN-Mason City IA-Austin MN,25, Omaha NE,24, Ottumwa IA-Kirksville MO,, St. Louis MO,100, Springfield MO,95, Columbia-Jefferson City MO,93, Kansas City MO,87, Joplin MO-Pittsburg KS,47, Paducah KY-Cape Girardeau MO-Harrisburg-Mount Vernon IL,35, Quincy IL-Hannibal MO-Keokuk IA,12, St. Joseph MO,, Ottumwa IA-Kirksville MO,, Eyelid, Itch, Therapy, Atropine, Jonesboro AR,100, Little Rock-Pine Bluff AR,58, Ft. Smith-Fayetteville-Springdale-Rogers AR,56, Shreveport LA,13, Springfield MO,8, Monroe LA-El Dorado AR,7, Memphis TN,5, Jonesboro AR,100, Little Rock-Pine Bluff AR,58, Ft. Smith-Fayetteville-Springdale-Rogers AR,56, Shreveport LA,13, Springfield MO,8, Monroe LA-El Dorado AR,7, Memphis TN,5, Lake Charles LA,100, New Orleans LA,72, Baton Rouge LA,67, Lafayette LA,64, Alexandria LA,61, Monroe LA-El Dorado AR,55, Shreveport LA,36, Bimatoprost,Breakout, Moxifloxacin,Breakout, Timolol, Lake Charles LA,100, New Orleans LA,72, Baton Rouge LA,67, Lafayette LA,64, Alexandria LA,61, Monroe LA-El Dorado AR,55, Shreveport LA,36, Wausau-Rhinelander WI,100, La Crosse-Eau Claire WI,72, Green Bay-Appleton WI,72, Milwaukee WI,64, Madison WI,63, Duluth MN-Superior WI,4, Minneapolis-St. Paul MN,3, Polymyxin B,Breakout, Dexamethasone,Breakout, Cat\’s eye,Breakout, Loteprednol,Breakout, Ciprofloxacin, Ofloxacin, Peoria-Bloomington IL,100, Champaign & Springfield-Decatur IL,92, Chicago IL,92, Quincy IL-Hannibal MO-Keokuk IA,88, Rockford IL,76, Davenport IA-Rock Island-Moline IL,61, Paducah KY-Cape Girardeau MO-Harrisburg-Mount Vernon IL,57, St. Louis MO,25, Evansville IN,3, Terre Haute IN,, Columbus-Tupelo-West Point MS,100, Hattiesburg-Laurel MS,88, Biloxi-Gulfport MS,85, Greenwood-Greenville MS,79, Jackson MS,76, Memphis TN,17, Meridian MS,, Baton Rouge LA,, Terre Haute IN,100, Indianapolis IN,95, South Bend-Elkhart IN,95, Ft. Wayne IN,84, Evansville IN,59, Louisville KY,29, Chicago IL,5, Cincinnati OH,3, Lafayette IN,, Bowling Green KY,100, Lexington KY,74, Louisville KY,58, Charleston-Huntington WV,29, Evansville IN,27, Paducah KY-Cape Girardeau MO-Harrisburg-Mount Vernon IL,17, Cincinnati OH,7, Knoxville TN,4, Nashville TN,4, Tri-Cities TN-VA,4, Cataract surgery , Knoxville TN,100, Nashville TN,79, Jackson TN,77, Tri-Cities TN-VA,69, Chattanooga TN,65, Memphis TN,63, Gentamicin,+190%, Huntsville-Decatur (Florence) AL,100, Montgomery (Selma) AL,84, Birmingham AL,83, Dothan AL,75, Columbus GA,60, Mobile AL-Pensacola (Ft. Walton Beach) FL,42, Meridian MS,, Dayton OH,100, Cleveland-Akron (Canton) OH,85, Columbus OH,80, Toledo OH,79, Youngstown OH,75, Cincinnati OH,66, Wheeling WV-Steubenville OH,43, Parkersburg WV,37, Lima OH,35, Charleston-Huntington WV,23, Ft. Wayne IN,2, Zanesville OH,, Pilocarpine,Breakout, Saline, similasan eye drops, Dayton OH,100, Cleveland-Akron (Canton) OH,85, Columbus OH,80, Toledo OH,79, Youngstown OH,75, Cincinnati OH,66, Wheeling WV-Steubenville OH,43, Parkersburg WV,37, Lima OH,35, Charleston-Huntington WV,23, Ft. Wayne IN,2, Zanesville OH,, Bluefield-Beckley-Oak Hill WV,100, Charleston-Huntington WV,70, Clarksburg-Weston WV,67, Parkersburg WV,62, Wheeling WV-Steubenville OH,36, Washington DC (Hagerstown MD),2, Harrisonburg VA,, New York,100, New Jersey,99, New Hampshire,93, West Virginia,92, Arkansas,91, Tennessee,90, Alabama,89, Florida,89, Kansas,89, Texas,88, Virginia,87, South Carolina,87, Arizona,87, Louisiana,87, California,86, North Carolina,85, Kentucky,85, Connecticut,84, Nebraska,83, Maryland,83, Oklahoma,83, Michigan,82, Illinois,82, Georgia,82, Utah,81, Ohio,81, Nevada,81, Rhode Island,80, Pennsylvania,80, Wyoming,79, South Dakota,79, New Mexico,78, Missouri,78, Iowa,78, Hawaii,78, Mississippi,76, Colorado,75, Massachusetts,74, North Dakota,74, Indiana,73, Montana,73, Idaho,73, Washington,73, Oregon,73, Minnesota,72, Wisconsin,72, Maine,71, Delaware,68, Alaska,65, District of Columbia,53, Vermont,52, dry eye drops,100, best eye drops,97, pink eye eye drops,68, pink eye drops,68, systane,65, systane eye drops,64, pink eye,63, eye allergy drops,63, refresh,54, refresh eye drops,54, eye drops for dry eyes,51, lumify,44, lumify eye drops,42, pataday eye drops,40, red eye drops,39, eye drops for dogs,38, pataday,37, dog eye drops,35, over the counter eye drops,35, eye drops for pink eye,32, best dry eye drops,30, glaucoma eye drops,29, cvs eye drops,29, prednisolone eye drops,27, antibiotic eye drops,27, olopatadine eye drops otc,Breakout, pataday eye drops cvs,”+1,000%”, chloramphenicol eye drops,+500%, blink tears lubricating eye drops,+500%, refresh relieva eye drops,+450%, tobramycin eye drops for dogs,+350%, flarex eye drops,+350%, naphcon a eye drops,+300%, betimol eye drops,+250%, sananga eye drops,+250%, travoprost eye drops,+250%, allergy eye drops for kids,+250%, polymyxin b tmp eye drops,+250%, kids allergy eye drops,+250%, refresh tears lubricant eye drops,+200%, polyvinyl alcohol,+200%, zioptan eye drops,+190%, prolensa eye drops,+180%, pataday eye drops,+180%, ocuflox eye drops,+170%, liquid msm eye drops,+160%, inveltys eye drops,+140%, xiidra eye drops,+140%, polyvinyl alcohol eye drops,+130%, contact safe eye drops,+130%, Richmond-Petersburg VA,100, Charlottesville VA,85, Roanoke-Lynchburg VA,79, Harrisonburg VA,76, Norfolk-Portsmouth-Newport News VA,71, Washington DC (Hagerstown MD),42, Tri-Cities TN-VA,27, Bluefield-Beckley-Oak Hill WV,, Macon GA,100, Albany GA,67, Atlanta GA,60, Augusta GA,57, Savannah GA,43, Columbus GA,30, Tallahassee FL-Thomasville GA,29, Chattanooga TN,18, Dothan AL,9, Jacksonville FL,3, Tampa-St. Petersburg (Sarasota) FL,100, Ft. Myers-Naples FL,99, West Palm Beach-Ft. Pierce FL,95, Orlando-Daytona Beach-Melbourne FL,93, Miami-Ft. Lauderdale FL,89, Jacksonville FL,87, Panama City FL,86, Gainesville FL,84, Tallahassee FL-Thomasville GA,62, Mobile AL-Pensacola (Ft. Walton Beach) FL,58, Artificial tears,Breakout, Tobramycin / Dexamethasone,Breakout, Bausch + Lomb Soothe XP-Xtra Protection,Breakout, Nasal spray,Breakout, Belladonna,Breakout, Expiration date,Breakout, Meibomian gland,Breakout, Trimethoprim,Breakout, LASIK,Breakout, Ilevro,Breakout, Refresh Plus,Breakout, Walmart Vision & Glasses,Breakout, Prednisolone acetate,Breakout, Erythema,Breakout, Chloramphenicol,Breakout, Flurbiprofen,Breakout, Hydrocortisone,Breakout, Tears,+200%, Ofloxacin,+160%, Timolol,+120%, Itch,+90%, Ketotifen,+80%, Prednisolone,+60%, Olopatadine,+60%, Adverse effect,+40%, Johnstown-Altoona PA,100, Wilkes Barre-Scranton PA,87, Harrisburg-Lancaster-Lebanon-York PA,81, Pittsburgh PA,78, Erie PA,68, Philadelphia PA,55, Elmira NY,26, Youngstown OH,13, Buffalo NY,1, TheraTears,Breakout, Prednisone,Breakout, Ocusoft Retaine MGD Complete Dry Eye Relief,Breakout, Artificial tears,Breakout, Lens,Breakout, Prescription drug,Breakout, Alrex,Breakout, Atropine,Breakout, Saline,Breakout, Corneal abrasion,Breakout, Eyewash,Breakout, Gland,Breakout, SYSTANE,+900%, Side effect,+350%, Visine,+250%, Itch,+250%, Preservative,+250%, Prednisolone,+250%, Pharmaceutical drug,+200%, Tears,+130%, Eye infection,+130%, Olopatadine,+120%, Ofloxacin,+50%, Over-the-counter drug,+40%, Eye,100, Eye drop,95, Drop,77, Dry eye syndrome,16, Dry eye,15, Allergy,10, Contact lens,8, Conjunctivitis,4, Preservative,4, Olopatadine,4, Prednisolone,3, Bausch + Lomb LUMIFY,3, Lens,3, Tears,3, Refresh,2, Ofloxacin,2, Ketotifen,2, Lubricant,2, Over-the-counter drug,2, Visine,2, Antihistamine,2, Infection,2, Cornea,2, Itch,2, Adverse effect,2, Cornea,Breakout, Can-C,Breakout, TheraTears,Breakout, Corneal abrasion,Breakout, Dorzolamide,Breakout, Can-C Lubricant Eye-Drops with Antioxidant N-Acetylcarnosine,Breakout, Dorzolamide / Timolol,Breakout, ICD-10,Breakout, Prescription drug,Breakout, TheraTears Lubricant Eye Drops,Breakout, Ocusoft Retaine MGD Complete Dry Eye Relief,Breakout, Dryness,Breakout, Refresh Tears,Breakout, Systane Preservative-Free Eye Drops,Breakout, Tacrolimus,Breakout, Macular degeneration,Breakout, Cetirizine,Breakout, Cromoglicic acid,Breakout, Fluorometholone,Breakout, Ciprofloxacin/dexamethasone,Breakout, Macula of retina,Breakout, Glaucoma surgery,Breakout, Lens,+150%, Preservative,+130%, Dry eye,+120%, dry eye drops,100, best eye drops,81, systane eye drops,49, allergy eye drops,47, pink eye drops,42, eye drops for dry eyes,38, refresh eye drops,31, prednisolone eye drops,30, lubricant eye drops,27, eye drops for dogs,25, zaditor eye drops,25, visine eye drops,25, eye drops for allergies,24, ofloxacin eye drops,24, eye drops for pink eye,24, best eye drops for dry eyes,22, preservative free eye drops,20, pataday eye drops,20, numbing eye drops,18, timolol eye drops,13, rohto eye drops,13, azelastine eye drops,13, antihistamine eye drops,13, restasis eye drops,11, ciprofloxacin,9, restasis eye drops,Breakout, zerviate eye drops,Breakout, rohto eye drops,+500%, numbing eye drops,+350%, moxifloxacin eye drops,+350%, pataday eye drops,+200%, timolol eye drops,+200%, eye drops for allergies,+190%, best eye drops for dry eyes,+160%, lubricant eye drops,+120%, lumigan eye drops,+110%, eye drops for dry eyes,+100%, dry eye drops,+100%, preservative free eye drops,+90%, prednisolone eye drops,+80%, zaditor eye drops,+70%, systane eye drops,+60%, best eye drops,+50%, eye drops for dogs,+50%, visine eye drops,+50%, azelastine eye drops,+50%, Burlington VT-Plattsburgh NY, Springfield-Holyoke MA,100, Boston MA-Manchester NH,82, Providence RI-New Bedford MA,26, Hartford & New Haven CT, Boston MA-Manchester NH,100, Burlington VT-Plattsburgh NY,65, Portland-Auburn ME,43, Bangor ME,100, Portland-Auburn ME,92, Presque Isle ME,
For search engines
Eye treatments. Vision improvements. Eyes, Researches, The science, Scientific breakthroughs in the world, Lenses, Glaucoma, Age-related macular degeneration, Dry eye syndrome (DES), Non-infectious uveitis. Cataract Treatment. Cataract. Best Eye Treatment Google Scholar PubMed Scopus Web of Science ScienceDirect Baidu SciELO EBSCOHost doaj Directory of Open Access Journals Academia Springer Science+Business Media Elsevier Thieme Medical Publishers ResearchGate Open Library Wiley Online Library Palgrave Macmillan Cambridge University Press Oxford University Press Routledge Axel Springer SE Emerald Group Publishing Princeton University Press Yale University Press MIT Press Academic Press Taylor and Francis CRC Press McGraw Hill Education Barnes and Noble Pearson Education BBC British Broadcasting Corporation Harvard University Random House SAGE Publications HarperCollins Imperial College London safe eye treatmen Ophthalmology surgery doctors Keywords:: Mitochondria Diabetic retinopathy Glaucoma Retinal degeneration Age-related macular degeneration duckduckgo startpage xueshu baidu f1000 com clinicalkey bionot scicrunch org neurosynth studies brainmaps org neuinfo babel eigenfactor latindex miar ub edu scimagojr com socialmention com med wanfangdata com cn viziometrics openi nlm nih.gov figshare proteinatlas berkeley sudoc redalyc lareferencia dialnet unirioja theses jstage erudit airitilibrary cnki muchong idref muckrack semanticscholar drugs com datamed drugbank informationisbeautiful uniprot chemweb brain-map binsearch doaj wellcomeopenresearch Biohackers Reddit ambulance Medicine
Tag Cloud:
Albania, Algeria, Andorra, Angola, Antigua and Barbuda, Argentina, Armenia, Australia, Austria, Azerbaijan, Bahamas, Bahrain, Bangladesh, Barbados, Belarus, Belgium, Belize, Benin, Bhutan, Bolivia, Bosnia and Herzegovina, Botswana, Brazil, Brunei, Bulgaria, Burkina Faso, Burundi, Côte d’Ivoire, Cabo Verde, Cambodia, Cameroon, Canada, Central African Republic, Chad, Chile, China, Colombia, Comoros, Congo (Congo-Brazzaville), Costa Rica, Croatia, Cuba, Cyprus, Czechia (Czech Republic), Democratic Republic of the Congo, Denmark, Djibouti, Dominica, Dominican Republic, Ecuador, Egypt, El Salvador, Equatorial Guinea, Eritrea, Estonia, Eswatini (fmr. “Swaziland”), Ethiopia, Fiji, Finland, France, Gabon, Gambia, Georgia, Germany, Ghana, Greece, Grenada, Guatemala, Guinea, Guinea-Bissau, Guyana, Haiti, Holy See, Honduras, Hungary, Iceland, India, Indonesia, Iran, Iraq, Ireland, Israel, Italy, Jamaica, Japan, Jordan, Kazakhstan, Kenya, Kiribati, Kuwait, Kyrgyzstan, Laos, Latvia, Lebanon, Lesotho, Liberia, Libya, Liechtenstein, Lithuania, Luxembourg, Madagascar, Malawi, Malaysia, Maldives, Mali, Malta, Marshall Islands, Mauritania, Mauritius, Mexico, Micronesia, Moldova, Monaco, Mongolia, Montenegro, Morocco, Mozambique, Myanmar (formerly Burma), Namibia, Nauru, Nepal, Netherlands, New Zealand, Nicaragua, Niger, Nigeria, North Korea, North Macedonia, Norway, Oman, Pakistan, Palau, Palestine State, Panama, Papua New Guinea, Paraguay, Peru, Philippines, Poland, Portugal, Qatar, Romania, Russia, Rwanda, Saint Kitts and Nevis, Saint Lucia, Saint Vincent and the Grenadines, Samoa, San Marino, Sao Tome and Principe, Saudi Arabia, Senegal, Serbia, Seychelles, Sierra Leone, Singapore, Slovakia, Slovenia, Solomon Islands, Somalia, South Africa, South Korea, South Sudan, Spain, Sri Lanka, Sudan, Suriname, Sweden, Switzerland, Syria, Tajikistan, Tanzania, Thailand, Timor-Leste, Togo, Tonga, Trinidad and Tobago, Tunisia, Turkey, Turkmenistan, Tuvalu, Uganda, Ukraine, United Arab Emirates, United Kingdom, United States of America, Uruguay, Uzbekistan, Vanuatu, Venezuela, Vietnam, Yemen, Zambia, Zimbabwe, USA, North America, South America, Europe
United States / USA
Alabama Alaska Arizona Arkansas California Colorado Connecticut Delaware Florida Georgia Hawaii Idaho Illinois Indiana Iowa Kansas Kentucky Louisiana Maine Maryland Massachusetts Michigan Minnesota Mississippi Missouri Montana Nebraska Nevada New Hampshire New Jersey New Mexico New York North Carolina North Dakota Ohio Oklahoma Oregon Pennsylvania Rhode Island South Carolina South Dakota Tennessee Texas Utah Vermont Virginia Washington West Virginia Wisconsin Wyoming
Alameda Albany Berkeley Dublin Emeryville Fremont Hayward Livermore Newark Oakland Piedmont Pleasanton San Leandro Union City Amador Ione Jackson Plymouth Sutter Creek Biggs Biggs Chico Gridley Oroville Paradise Angels Camp Colusa Williams Antioch Brentwood Clayton Concord Danville El Cerrito Hercules Lafayette Martinez Moraga Oakley Orinda Pinole Pittsburg Pleasant Hill Richmond San Pablo San Ramon Walnut Creek Crescent City Placerville South Lake Tahoe Clovis Coalinga Firebaugh Fowler Fresno Huron Kerman Kingsburg Mendota Orange Cove Parlier Reedley Sanger San Joaquin Selma Orland Willows Arcata Blue Lake Eureka Ferndale Fortuna Rio Dell Trinidad Brawley Calexico Calipatria El Centro Holtville Imperial Westmorland Bishop Arvin Arvin Bakersfield California City Delano Maricopa Mcfarland Ridgecrest Shafter Taft Tehachapi Wasco Avenal Corcoran Hanford Lemoore Clearlake Lakeport Susanville Agoura Hills Alhambra Arcadia Artesia Avalon Azusa Baldwin Park Bell Bellflower Bell Gardens Beverly Hills Bradbury Burbank Calabasas Carson Cerritos Claremont Commerce Compton Covina Cudahy Culver City Diamond Bar Downey Duarte El Monte El Segundo Gardena Glendale Glendora Hawaiian Gardens Hawthorne Hermosa Beach Hidden Hills Huntington Park Industry Inglewood Irwindale La Canada Flintridge La Habra Heights Lakewood La Mirada Lancaster La Puente La Verne Lawndale Lomita Long Beach Los Angeles Lynwood Malibu Manhattan Beach Maywood Monrovia Montebello Monterey Park Norwalk Palmdale Palos Verdes Estates Paramount Pasadena Pico Rivera Pomona Rancho Palos Verdes Redondo Beach Rolling Hills Rolling Hills Estates Rosemead San Dimas San Fernando San Gabriel San Marino Santa Clarita Santa Fe Springs Santa Monica Sierra Madre Signal Hill South El Monte South Gate South Pasadena Temple City Torrance Vernon Walnut West Covina West Hollywood Westlake Village Whittier Chowchilla Madera Belvedere Corte Madera Fairfax Larkspur Mill Valley Novato Ross San Anselmo San Rafael Sausalito Tiburon Fort Bragg Point Arena Ukiah Willits Atwater Dos Palos Gustine Livingston Los Banos Merced Alturas Mammoth Lakes Carmel-By-The-Sea Del Rey Oaks Gonzales Greenfield King City Marina Monterey Pacific Grove Salinas Sand City Seaside Soledad American Canyon Calistoga Napa St Helena Yountville Grass Valley Nevada City Truckee Aliso Viejo Anaheim Brea Buena Park Costa Mesa Cypress Dana Point Fountain Valley Fullerton Garden Grove Huntington Beach Irvine Laguna Beach Laguna Hills Laguna Niguel Laguna Woods La Habra Lake Forest La Palma Los Alamitos Mission Viejo Newport Beach Orange Placentia Rancho Santa Margarita San Clemente San Juan Capistrano Santa Ana Seal Beach Stanton Tustin Villa Park Westminster Yorba Linda Auburn Colfax Lincoln Loomis Rocklin Roseville Portola Banning Beaumont Blythe Calimesa Canyon Lake Cathedral City Coachella Corona Desert Hot Springs Eastvale Hemet Indian Wells Indio Jurupa Valley Lake Elsinore La Quinta Menifee Moreno Valley Murrieta Norco Palm Desert Palm Springs Perris Rancho Mirage Riverside San Jacinto Temecula Wildomar Citrus Heights Elk Grove Folsom Galt Isleton Rancho Cordova Sacramento Hollister San Juan Bautista Adelanto Apple Valley Barstow Big Bear Lake Chino Chino Hills Colton Fontana Grand Terrace Hesperia Highland Loma Linda Montclair Needles Ontario Rancho Cucamonga Redlands Rialto San Bernardino Twentynine Palms Upland Victorville Yucaipa Yucca Valley Carlsbad Chula Vista Coronado Del Mar El Cajon Encinitas Escondido Imperial Beach La Mesa Lemon Grove National City Oceanside Poway San Diego San Marcos Santee Solana Beach Vista San Francisco Escalon Lathrop Lodi Manteca Ripon Stockton Tracy Arroyo Grande Atascadero El Paso De Robles Grover Beach Morro Bay Pismo Beach San Luis Obispo Atherton Belmont Brisbane Burlingame Colma Daly City East Palo Alto Foster City Half Moon Bay Hillsborough Menlo Park Millbrae Pacifica Portola Valley Redwood City San Bruno San Carlos San Mateo South San Francisco Woodside Buellton Carpinteria Goleta Guadalupe Lompoc Santa Barbara Santa Maria Solvang Campbell Cupertino Gilroy Los Altos Los Altos Hills Los Gatos Milpitas Monte Sereno Morgan Hill Mountain View Palo Alto San Jose Santa Clara Saratoga Sunnyvale Capitola Santa Cruz Scotts Valley Watsonville Anderson Redding Shasta Lake Loyalton Dorris Dunsmuir Etna Fort Jones Montague Mount Shasta Tulelake Weed Yreka Benicia Dixon Fairfield Rio Vista Suisun City Vacaville Vallejo Cloverdale Cotati Healdsburg Petaluma Rohnert Park Santa Rosa Sebastopol Sonoma Windsor Ceres Hughson Modesto Newman Oakdale Patterson Riverbank Turlock Waterford Live Oak Yuba City Corning Red Bluff Tehama Dinuba Exeter Farmersville Lindsay Porterville Tulare Visalia Woodlake Sonora Camarillo Fillmore Moorpark Ojai Oxnard Port Hueneme Santa Paula Simi Valley Thousand Oaks Ventura Davis West Sacramento Winters Woodland Marysville Wheatland Las Vegas Henderson Reno North Las Vegas Paradise Spring Valley Sunrise Manor Enterprise Sparks Carson City Whitney Pahrump Winchester Summerlin South Sun Valley Elko Fernley Mesquite Spanish Springs Boulder City Spring Creek Gardnerville Ranchos Cold Springs Dayton Incline Village Fallon
Laughlin Winnemucca Moapa Valley Gardnerville Indian Hills Johnson Lane Lemmon Valley Silver Springs West Wendover Ely Battle Mountain Nellis AFB Minden Yerington Hawthorne Washoe Valley Kingsbury Carlin Tonopah Lovelock Stagecoac Sandy Valley Topaz Ranch Estates Smith Valley East Valley Bunkerville Pioche Mogul Ruhenstroth Golden Valley Verd Jackpot Panaca Owyhee Alamo Schurz Wells Round Hill Village Stateline McGill Caliente Indian Springs Beatty Wadsworth 792Virginia City Grass Valley Moapa Town Genoa Fish Springs Osino Eureka and Crescent Valley Carter Springs Fallon Station Zephyr Cove Mount Charleston Lakeridge Fort McDermitt Blue Diamond Dyer Skyland Searchlight Walker Lake Goldfield Sutcliffe Lamoille Double Spring Glenbrook Nixon Imlay Topaz Lake Crystal Bay Mina TIE Humboldt River Ranch and Silver City Silver Peak Empire Ruth McDermitt Austin Cal-Nev-Ari TIE Gabbs and Lund Kingston Valmy Golconda Rachel Orovada Hiko Montello Baker Paradise Valley Bennett Springs Oasis Logan Creek TIE Gerlach and Nelson Beaverdam Preston Mitotech Skq1 Vizomitin